Fig. 14.1
Acetaldehyde production by Streptococcus gordonii on the PBB-Schiff’s agar. (1) V2016wt; (2) ΔadhA; (3) ΔadhB; (4) ΔadhAB; (5) ΔadhE; (6) ΔadhAE; (7) ΔadhBE; (8) ΔadhABE
14.2.4 Alcohol Dehydrogenases of S. gordonii V2016
Because existing enzyme activity gel assays (zymograms) were not sensitive enough to detect multiple ADH activities from crude bacterial samples, we developed a more sensitive zymogram method, which allowed us to detect multiple ADHs simultaneously on the same gel with crude bacterial lysates. ADH and ALDH activities of the testing bacteria were determined by a specific zymogram method improved from several methods described previously [11, 34, 35]. Nitroblue tetrazolium (NBT) in the presence of phenazine methosulfate (PMS) reacts with nicotinamide adenine dinucleotide phosphate (NADPH) produced by dehydrogenases to produce an insoluble blue-purple formazan. This new NBT-PMS detection method was used to visualize ADH and ALDH in polyacrylamide gels [30]. Yeast ADH and ALDH (Sigma-Aldrich) were used as positive controls.
By knocking out three adh genes individually and in various combinations, we identified that S. gordonii V2016 has three primary ADHs, AdhA, AdhB, and AdhE (Fig. 14.2), which all recognized ethanol as a substrate with different activities (Fig. 14.3). Additionally, we have also identified a secondary ADH, S-AdhA, which specifically recognizes the secondary alcohol, 2-propanol, and a dehydrogenase specific for threonine (Fig. 14.4). These two dehydrogenases, however, do not recognize ethanol as their substrate (Figs. 14.2 and 14.3a), despite that AdhA recognizes both threonine and 2-propanol as its substrate (Fig. 14.4).
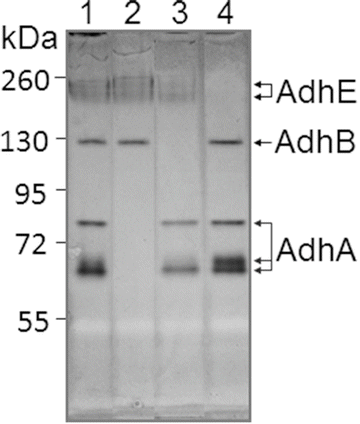
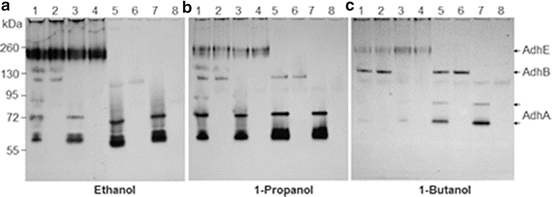
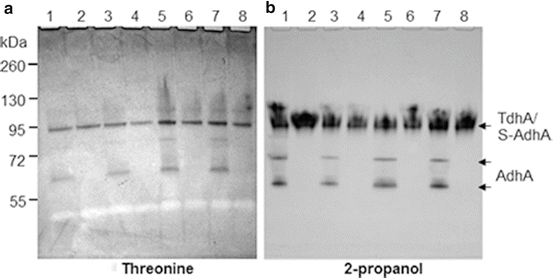
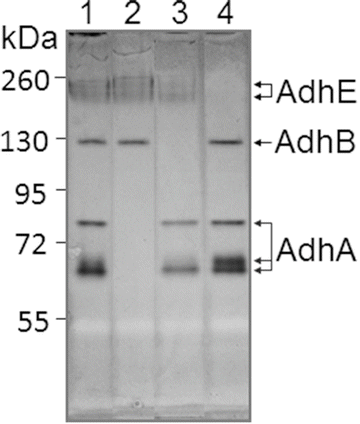
Fig. 14.2
Streptococcus gordonii V2016 ADH distribution analysis by zymogram without added Fe2+ and Zn2+: (1) V2016wt; (2) ΔadhA; (3) ΔadhB; (4) ΔadhE. Note: missing band(s) of each Δadh mutant indicates the location(s) of the target ADH
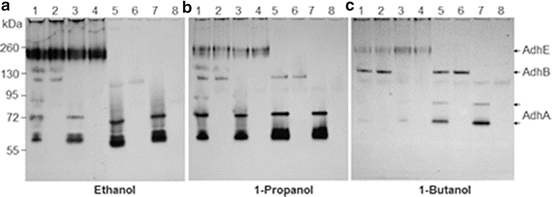
Fig. 14.3
Analysis of substrate preference of Streptococcus gordonii V2016 ADH. Fe2+ and Zn2+ were added to the growth medium and zymogram detection solution. (1) V2016wt; (2) ΔadhA; (3) ΔadhB; (4) ΔadhAB; (5) ΔadhE; (6) ΔadhAE; (7) ΔadhBE; (8) ΔadhABE. Note: in the wild type strain, AdhE prefers ethanol, AdhA prefers 1-propanol and AdhB prefers 1-butanol
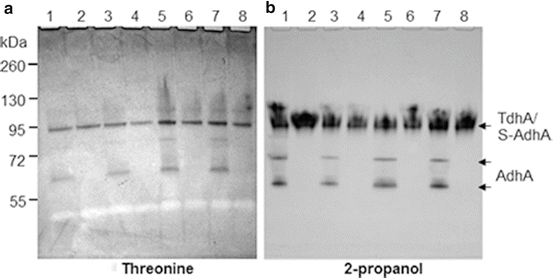
Fig. 14.4
Identification of two novel dehydrogenases in S. gordonii, the threonine dehydrogenase (TdhA) and the secondary alcohol dehydrogenase (S-AdhA) by zymograms. (1) V2016wt; (2) ΔadhA; (3) ΔadhB; (4) ΔadhAB; (5) ΔadhE; (6) ΔadhAE; (7) ΔadhBE; (8) ΔadhABE. Note: AdhA reacted with both threonine and 2-propanol because mutants with ΔadhA did not show these bands
In addition to S. gordonii V2016, three other S. gordonii strains including V288, CHI, and 110-3, and five S. sanguinis laboratory strains including 133-79, S7, Blackburn, SK36 and ATCC 10556, and 11 oral Streptococcus isolates, including four strains produced only acetaldehyde, two strains produced both acetic acid and acetaldehyde, and five strains produced only acetic acid from ethanol (see legend to Fig. 14.5 for strain names), were also analyzed for both ADH and ALDH activities.
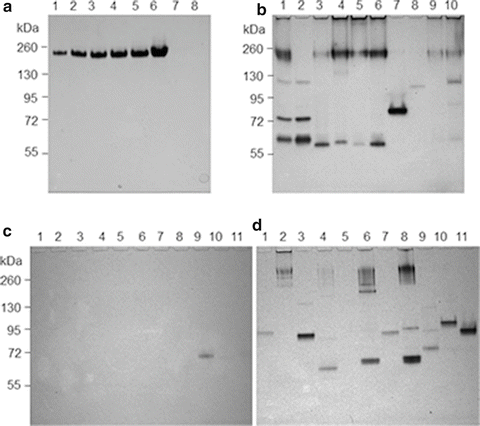
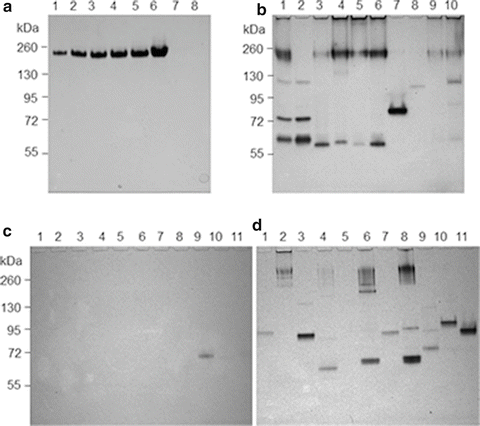
Fig. 14.5
(a) ALDH zymogram: 1-6, Saccharomyces sereviciae ALDH controls: 1, 0.1U; 2, 0.25U; 3, 0.5U; 4, 0.75U; 5, 1 U; 6, 3 U; 7, blank; 8, S. gordonii V2016wt. (b) ADH zymogram: 1-3, S. gordonii V2016wt, V2016ΔadhE and V288; 4 and 5, S. sanguinis S7 and Blackburn; 6 and 7, S. gordonii CHI and 110-3; 8-10, S. sanguinis 133-79, SK36 and ATCC 10556 (ALDH zymogram of these strains was all negative; data not shown). (c) ALDH zymogram: 1-4 (produced only acetaldehyde from ethanol), S. salivarius 101-1; S. sanguinis 104-5; S. salivarius 109-2, and S. sanguinis 109-3; 5 and 6 (produced both acetaldehyde and acetic acid from ethanol), S. oralis 108 and S. mitis 110-5; 7-11 (produced only acetic acid from ethanol), S. salivarius 101-7, S. mitis 104-4, S. salivarius 107-2, 110-1, and 110-4. (d) ADH zymogram of the same 11 strains displayed in (c). Note: only S. salivarius 107-2 displayed an ALDH band, which is different from AdhE. S. oralis 108 did not show detectable ADH
Among three ADHs in S. gordonii V2016, a cross regulation of their activities may exist. As shown in Fig. 14.2, when the activity of AdhE is weak due to the lack of its cofactor Fe2+ or missing due to gene deletion, the activities of AdhA and AdhB were relatively strong. However, when Fe2+ and Zn2+ were supplemented to the growth medium, the AdhE activity became substantially increased (Fig. 14.3a, Lanes 1–4) but the activities of both AdhA and AdhB were suppressed, possibly by the increased activity of AdhE. However, when the adhE gene was deleted, the activity of AdhA was increased (Fig. 14.3a, Lanes 5, 7), but not AdhB, which appeared to be relatively independent from AdhE regulation. A similar scenario was also observed when 1-propanol (Fig. 14.3b) and 1-butanol were used as substrates (Fig. 14.3c). These results suggest that AdhE may be the major ADH in S. gordonii. When its activity is upregulated, the activities of other ADHs, especially the AdhA, are reduced.
The ADH activities of V2016 and its various adh mutants were analyzed with the NBT-PMS zymogram method. First, the approximate size of each ADH was estimated by testing each adh gene-deletion mutant against the wild type on an ADH zymogram. As shown in Fig. 14.2, the wild type V2016 displays all three functional ADH enzymes (Lane 1). The ∆adhA mutant lacks three bands between 55 and 72 kDa (Lane 2). The ∆adhB mutant misses a single band near 130 kDa (Lane 3). The ∆adhE mutant misses top two bands around 260 kDa (Lane 4). Because the actual molecular sizes of these enzymes cannot be determined by the native polyacrylamide gel the sizes and shapes of these enzymes can only be estimated. For example, since three bands are related AdhA, this enzyme may take three different forms (e.g., monomer, dimer, and/or trimer).
The DNA sequences of adhA and adhB both show a zinc-binding domain, but only AdhA showed enhanced ADH activity after zinc supplementation and only in the absence of AdhE (Fig. 14.3a). Supplementing iron significantly enhanced AdhE activity (Fig. 14.3a, Lanes 1–4) suggesting that the AdhE protein is an iron-dependent ADH. However, in mutants with adhE inactivation (Fig. 14.3, Lanes 5 and 7), the activity of AdhA is enhanced, but the activity of AdhB is not. The AdhB protein has apparent one (Fig. 14.2) or two conformations (Fig. 14.3). The second AdhB band showed up only when zinc was supplemented into the growth medium and when AdhE is present.
14.2.5 Absence of ALDH in S. gordonii V2016
The S. gordonii genomic data [31] showed that this bacterium has a gene (acdH, SGO_0113) encoding the putative dual functional ALDH/ADH (AdhE). It has homology to the ALDH/ADH dual function AdhE in other bacteria [36]. Therefore, it is important to test whether S. gordonii V2016 AdhE also has dehydrogenase activity for acetaldehyde. As shown in Fig. 14.5a, we tested S. gordonii V2016 with the optimized NBT-PMS zymogram. However, S. gordonii V2016 did not show any detectable ALDH activity (Fig. 14.5a, Lane 8). To make sure that this method is sensitive enough to detect microbial ALDH, we used S. cerevisiae ALDH as a positive control. The zymogram detected ALDH activity as low as 0.1 U. This method has also detected ALDH from another oral Streptococcus strain, S. salivarius 107-2 (Fig. 14.5c). Therefore, the zymogram method should be reliable and the negative result indicated that S. gordonii V2016, as well as other tested oral Streptococcus strains, did not have a detectable ALDH. Because the adhE gene of these oral streptococci is highly homologous to adhE in other bacteria [36] that encodes a bifunctional ALDH/ADH, there might be a mutation(s) in its ALDH domain. This finding, together with the finding of oral Neisseria [11], indicates that genetic polymorphisms in ALDH in bacteria may exist similar to that seen in humans [21, 37]. Because most tested oral streptococcal strains showed multiple ADH, but no ALDH, the enzyme distribution bias may contribute to their excessive production of acetaldehyde from ethanol.
In the East Asian population of humans, a rather high percentage (up to 30 %) carries a defective ALDH2, which is caused by a point mutation resulting in a Glu to Lys substitution at the amino acid position 487, and is referred to as ALDH2*487Lys (previous symbol: ALDH2*2) [38, 39]. In this study, we observed that in most strains of oral Streptococcus tested, the AdhE protein has only ADH but no ALDH activity. This is also true in Neisseria [11]. This indicates that the adhE gene of these bacteria might have lost its ability to express functional ALDH during the course of evolution. Based on a recent study on bacterial evolution [40], if a gene is nonessential for bacterial survival, more mutations can be accumulated in comparison with genes that are essential. Because adhE is nonessential, a random mutation in adhE could be allowed and be passed down to the offspring. The questions are how many bacterial species carry such a mutation in their adhE gene and which base substitution(s) may inactivate its ALDH activity.
14.2.6 Substrate Specificities of S. gordonii ADHs
In addition to ethanol, we also tested other alcohols, including methanol, 1-propanol, 2-propanol, 1-butanol, and tertiary-butanol, and the amino acid threonine with the NBT-PMS zymogram method. Except for methanol and tertiary-butanol which showed no activity all other tested substrates showed varied activities with these three primary ADHs (Figs. 14.3 and 14.4). The preferred substrates for AdhA, AdhB, and AdhE were 1-propanol, 1-butanol, and ethanol, respectively. Additionally, two new dehydrogenases for threonine and 2-propanol were observed. Insertion-inactivation study showed that the dehydrogenase encoded by the gene located at SGO_0440 was specific for threonine. We therefore named this gene tdhA, encoding the threonine dehydrogenase. To find the gene locus coding for 2-propanol dehydrogenase, we identified three genes with homologies to major dehydrogenases: SGO_0273, SGO_0440, and SGO_0841. However, none of the mutations in the three loci had inactivated the enzyme activity for 2-propanol. Therefore, the gene encoding the dehydrogenase specific for the secondary alcohol remains to be determined. The specificities of five dehydrogenases to various tested substrates are listed in Table 14.1. The substrate specificity analysis showed that three ADHs of S. gordonii V2016 all recognize a broad range of substrates besides ethanol, but the activities of S-AdhA and TdhA were quite specific to their preferred substrates. It appears to be disadvantageous for a bacterium to have multiple ADHs that all produce the toxic metabolite from ethanol. Having multiple different ADHs may offer the bacterium competitive growth advantage in the environment due to the capability of utilizing multiple different nutrient substrates.
Table 14.1
Substrate specificity of S. gordonii V2016 dehydrogenases
Substrate | AdhA | AdhB | AdhE | S-AdhA | TdhA |
---|---|---|---|---|---|
Acetaldehyde | − | − | − | − | − |
Methanol | − | − | − | − | − |
Ethanol | +a | + | +++ | − | − |
1-Propanol | +++ | + | + | − | − |
2-Propanol | + | − | − | +++ | − |
1-Butanol | + a | +++ | + | ± | − |
tert-Butanol | − | − | − | − | − |
Threonine | + | ± | − | − | ++ |
14.2.7 ADH/ALDH Profiles Vary Among Strains of Oral Streptococcus
As shown in Fig. 14.5b, among four S. gordonii strains tested, only V2016 showed all three ADHs (AdhA, AdhB, and AdhE). S. gordonii V288 and CHI showed AdhA and AdhE, but no AdhB. However, S. gordonii 110-3 showed only one ADH similar to AdhA. Among five S. sanguinis strains tested, S7 and Blackburn showed only AdhA and AdhE. 133-79 showed only a weak AdhB. SK36 showed only a weak AdhE. Although ATCC 10556 showed four bands representing AdhA, AdhB, and AdhE, their activities are relatively weak. As shown in Figs. 14.5c, d, three groups of oral Streptococcus strains included four strains produced only acetaldehyde, two strains produced both acetic acid and acetaldehyde, and five strains produced only acetic acid from ethanol were tested for both ALDH and ADH. Although most strains showed one or more ADHs, only one strain, S. salivarius 107-2 showed an ALDH activity band, which is significantly smaller than AdhE (Fig. 14.5c). Because its size is not within the range of AdhE, it may be a novel ALDH (Table 14.2).
Table 14.2
Furin expression associated with Streptococcus adherence to immortalized oral keratinocytes (hTERT HOK)
Cell | % of Cells express furind | % of Cell with ≥10 bacteria adhered to surfaced |
---|---|---|
Untreated hTERT HOK control | 22 | ND |
101-7 + 1 % ethanola | 45 | 75 |
101-7 + 1 % ethanol + CMKb | 28 | 75 |
101-7 | 42 | 72 |
101-1 + 1 % ethanolc | 66 | 74 |
101-1 + 1 % ethanol + CMK | 46 | 31 |
101-1 | 37 | 43 |
With a broad range of substrate preferences and varied ADH profiles, these bacteria may metabolize ethanol differently. Because several acetic acid producers did not show ALDH activity bands, these bacteria may have either very weak ALDH or use different mechanisms to produce acetic acid from ethanol. In addition to enzymatic pathways, ethanol can also be oxidized by non-enzymatic free radical pathways to produce acetaldehyde [41, 42]. This might explain S. oralis 108 that showed no detectable ADH activity (Fig. 14.5d) but still produced excessive acetaldehyde from ethanol.
AdhE is highly conserved and may have multiple functions depending upon different bacterial species. For example, in Leuconostoc, AdhE is a bifunctional ALDH/ADH [36]. In Escherichia coli [43] and Streptococcus bovis [44], AdhE has three distinct enzymatic activities: ADH, acetaldehyde-CoA dehydrogenase, and pyruvate formate-lyase (PFL) deactivase. In Listeria, AdhE is also a major adhesion protein (named LAP, stands for Listeria-adhesion protein) and is located on the cell surface [45]. In Thermoanaerobacter mathranii, AdhE is a bifunctional ALDH/ADH responsible for ethanol production [46].
14.2.8 Effect of adh Deletions on Bacterial Growth in Medium Containing Ethanol or Acetaldehyde
The V2016 wild type and its seven mutants, ΔadhA, ΔadhB, ΔadhE, ΔadhAB, ΔadhAE, ΔadhBE, and ΔadhABE, were analyzed for growth in THY broth (control) and THY broth supplemented with 1 % ethanol or 1 % acetaldehyde. Each strain was grown in three tubes of 5 mL THY broth overnight with serial diluted inoculations. In the second morning, the culture at mid-exponential phase was transferred with a 1:100 dilution to the three different testing media and incubated at 37 °C. The optical density at 600 nm was measured every 30 min with a Genesys 20 Spectrophotometer. To better present the bacterial growth data, optical density readings as a function of time in the logarithmic growth phase were converted to doubling time (Fig. 14.6).
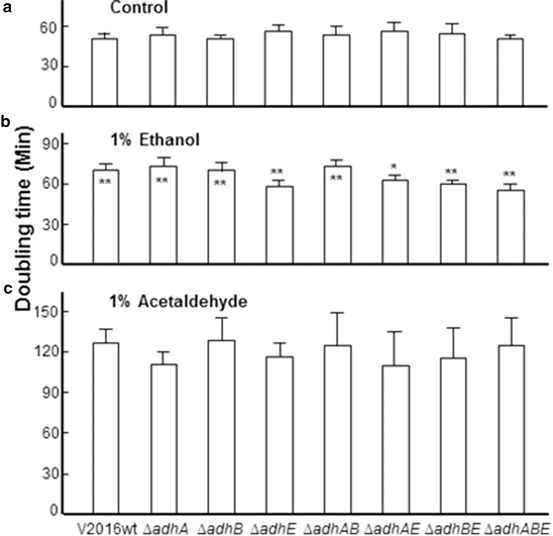
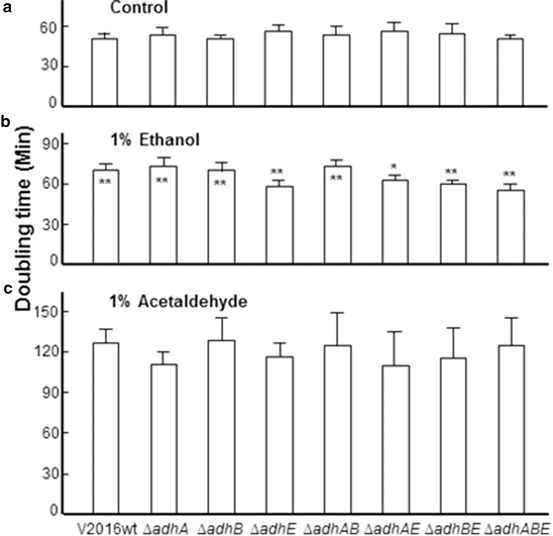
Fig. 14.6
Doubling times of Streptococcus gordonii V2016wt and seven Δadh mutants grown in THY or THY containing 1 % ethanol or 1 % acetaldehyde. Asterisk represents statistic difference by the Student’s t-test (*p < 0.05; **p < 0.01). When asterisk is on top of the data bar, it represents significant difference between the doubling time of this strain and its wild type growing in the same medium. When asterisk is inside the data bar, it represents significant difference between the same strain growing in THY and THY containing 1 % ethanol. All strains growing in THY containing 1 % acetaldehyde had significantly longer doubling times than grown in other media. Each data bar represents the average of five measurements plus standard deviation
Deletion of any of these three adh genes did not show apparent difference in the growth doubling times in THY without supplemented ethanol. However, when ethanol was supplemented at 1 %, the growth became slowed with significantly longer doubling times for the wild type and the ΔadhA and/or ΔadhB mutants. The four mutants containing adhE deletion had largely the same doubling times between growth in THY alone and THY supplemented with 1 % ethanol. In comparison with the wild type, these four mutants showed significantly shorter doubling times in THY supplemented with 1 % ethanol. All eight strains displayed significantly longer doubling times in THY supplemented with 1 % acetaldehyde.
The significant increase in bacterial doubling time of all eight strains indicates that acetaldehyde is more toxic than ethanol. A similar effect is also reported in a study with yeast [47]. Therefore, mutants that lack the enzyme for the production of acetaldehyde can be more tolerant to ethanol than the wild type [5]. The growth study (Fig. 14.6) showed that all four mutants containing ∆adhE when grown in THY containing 1 % ethanol had no significant increase in doubling times comparing with growth in control THY. However, the ΔadhA and/or ΔadhB mutants showed increased doubling times like the wild type when grown in THY containing 1 % ethanol. This suggests that AdhA and AdhB may be less involved in acetaldehyde production from ethanol than AdhE in S. gordonii V2016.
14.3 Ethanol Metabolism by Oral Streptococci Increases Bacterial Adhesion, HPV Entry, and HPV-Mediated Malignant Transformation of Oral Keratinocytes
Because both streptococci and HPV are commonly found in the oropharynx at relatively high levels and both independently produce damages to HOK, we hypothesized that bacteria and HPV may cooperate to affect oral mucosal cells and increase the risk for malignant transformation. When encountering environmental stress, such as exposure to ethanol, bacteria may increase attachment to the oral mucosa. This may disrupt the wellbeing of oral mucosal cells due to release of virulence factors and/or ethanol metabolites by the attached bacteria. As a result, oral mucosal cells may become vulnerable to viral infection or undergo virus-mediated malignant changes for cells already infected by a virus.
14.3.1 Ethanol Metabolites Production by Bacteria and Toxicity to Keratinocytes
We tested the cytotoxicity of ethanol and its metabolites, acetaldehyde and malondialdehyde, produced by bacteria. Even a low level of ethanol exposure could result in a loss of normal physiologic function such as oxidation metabolism in keratinocytes. This cell feature was examined in the presence of Streptococcus mutans and exposure to different levels of ethanol. We expected to find a low concentration of ethanol that enhanced the attachment of S. mutans to HOK but did not alter oxidative metabolism of the cells. This was accomplished by incubation of HOK (HPV/HOK-16B) and S. mutans together for 24 h in the cell medium exposed to increasing levels of ethanol (0.1, 1, 10, 20%, v/v). We used 5-carboxyfluorescein diacetate (5-CFDA) (Calbiochem, La Jolla, CA), which requires a viable metabolic active cell, to characterize oxidative stress using a microplate reader (excitation, 495 nm; emission, 520 nm) to provide a determination of keratinocyte viability. Acetaldehyde concentration (in mM) was also recorded with a spectrophotometric analysis.
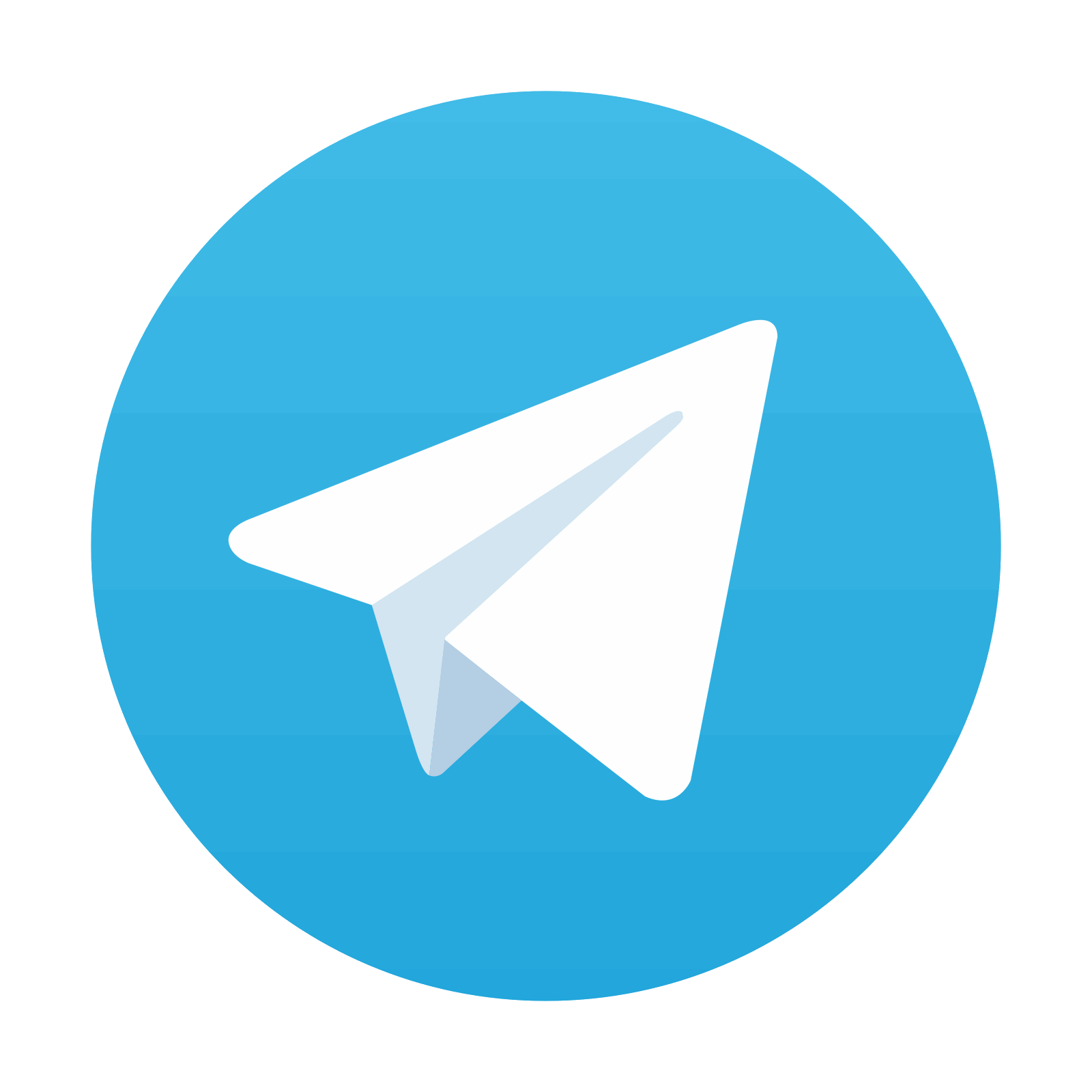
Stay updated, free articles. Join our Telegram channel

Full access? Get Clinical Tree
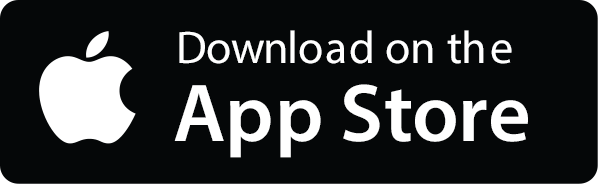
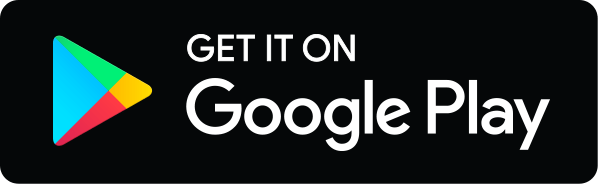