Is there Physiological Ageing for the Brain?
From an evolutionist point of view, a compelling question is what is the advantage for our species to have a prolonged, senescent period of life in which women cease to be reproductive? Notwithstanding culture-based influences that provide senior members of a group with a protected and honoured social status, it has been proposed that elderly individuals, especially grandmothers, could play a specific role in the transmission of knowledge concerning foraging and other context-dependent hints and strategies crucial to individual and social survival. Therefore, it is conceivable, on biological grounds, that non-reproductive, ageing individuals who can communicate, over a significant period, their experience to junior congeners and participate in the (prolonged) education of children represent an evolutionarily advantage.2 From a physiological perspective, it should be remarked that the maintenance of the large and complex human brain supporting efficient, long-lived episodic memory implies especially high energy consumption as the brain’s energetic needs relative to the total body metabolism is remarkably high in humans (∼20% of the body energy consumption). The intimate basis of this enormous energy need probably relates to (i) the considerable membrane surface of most neurons whose dendritic and axonal arborescence extend very far from the cell body and (ii) the energetic cost of molecular trafficking and ionic exchanges throughout membranes of the ‘neural tree’. It should be noted that some regions of the consciousness-related ‘default mode’ network, such as the posterior cingulate cortex, also support high-level autonoetic memory representations. It might not be pure coincidence that this region is also characterized by both a tight coupling between oxidative energy consumption and synaptic firing3 and heavy binding of amyloid-specific ligands at early stages of AD.4, 5 Further, the effect of constant learning and maintenance of long-term memory during ageing have to relate also to morphological plasticity in the brain. A very slight, non-linear and brain-wide trend to grey matter thinning with preponderance in the parietal and frontal convexity is observed in normal subjects from the second to the eighth decade; this slight frontal atrophy is associated with a decrease in performance on executive and working memory tests.6 By contrast, the evolution of white matter volume proceeds differently over decades, with a slight growth trend from the third to the sixth decade followed by a rapid decline with further ageing;7 white matter abnormalities in magnetic resonance imaging (MRI), typically small hyperintensities in the periventricular regions, increase with age and represent an independent risk factor for dementia and stroke.8
At the microscopic level, the estimation of the reduction in the density of neurons in the cortex has led to controversies; differences between cortical territories might account for these discrepancies, but some moderate, age-related decrease in branching in the dendritic tree and the size of neurons has been more consensually acknowledged (for a review, see Burke and Barnes9). Interestingly, normal ageing seems to relate to a shrinkage of cortical mini-columns,10 although adaptive plasticity may also lead to longer terminal segments dendritic trees11 in addition to new synapses and neo-neurogenesis12 that might offset the effects of disappeared connections. At the molecular level, neural plasticity depends on a variety of mechanisms, among which one should note the role of immediate early genes (CJun, Cfos and Zif238), in addition to a number of other pathways involved in axonal growth, cytoskeletal assembly/transport, signalling and lipogenic/uptake pathways, according to Rowe et al.,13 who also described upregulation in immune/inflammatory, lysosomal, lipid/protein degradation, cholesterol transport, transforming growth factor and cAMP signalling pathways. The influence of education and sustained, challenging cognitive activities on brain structure and functions has been described as the ‘cognitive reserve hypothesis’ by Stern et al.;14 such an influence of cognition-related neural activities over the lifespan would also elicit brain resilience to pathology. Although the existence of learning-related brain plasticity (from cellular to gyral morphology levels) is beyond dispute,15 these effects across subject groups differing in education have not been unequivocally confirmed and the protective mechanisms of ‘cognitive reserve’ against brain pathologies are still poorly understood.
Pathological Ageing: Proposed Cellular and Molecular Mechanisms
The challenging need for maintenance over decades of high energy consumption in the hugely complex cellular brain architecture has been mentioned above; in the context of such human-specific physiological condition, a number of mechanisms that would be at the origin of ageing-related degenerative diseases have been put forward, especially a lack of clearance of toxic metabolic by-products, oxidative stress and disturbed Ca2+ homeostasis. A number of neurodegenerative diseases are associated with the aggregation of misfolded constitutive cellular proteins such as tau, α-synuclein and β-amyloid.16 The last protein has attracted considerable attention as a consequence of its major implication in the most frequent, age-related degenerative brain disease, AD.
The specific role of the β-amyloid protein in its various monomeric, oligomeric and aggregated or fibrillary forms in the pathophysiology of AD remains to be elucidated, in addition to the mechanistic link between β-amyloid accumulation and pathological accumulation of the hyperphosphorylated form of tau protein in neuronal microtubules. The aggregated, mature form of β-amyloid constitutes the core of senile plaques observed in the brain of AD patients and also in normal elderly subjects, although to a far lesser extent; this typical neuropathological feature probably represents the final state of the pathology process rather than the origin of neuronal death in AD. Oligomeric, soluble β-amyloid has been shown to have toxic effect at the synaptic level via overstabilization of mGlu5 receptors provoking excessive intracellular calcium influx;17 damage to synapses seems to be one of the earliest lesion stages in pathological ageing with synaptic loss located in key regions such as the mesial temporal cortex.18 The chronic activation of pro-inflammatory pathways eliciting glial cell activation has been outlined as one of the likely basic mechanisms leading to cell death in neurodegenerative diseases, and even β-amyloid has been related to such inflammatory pathways, putatively activated to fight microbial diseases.19 One of the only established important genetic risk factors in the late-onset forms of AD is genotype epsilon4/epsilon4 of the ApoE protein, while ApoE3 has protective influence for cardiovascular diseases in ageing. In evolutionistic terms, ApoE4 would be an old form whereas ApoE3 seems to have been selected later, perhaps as a consequence of the changes in the human diet when it went on to involve more and more animal fat. ApoE has a major role in the transport of cholesterol and elevated values of cholesterol blood concentration also represent a risk for AD. A direct binding mechanism has recently been suggested between cholesterol and the C-terminal portion of the amyloid precursor protein.20
At the intracellular level, the key role of Ca2+ in a number of metabolic processes, from energy production to gene regulation, consists in a transient influx of calcium in the cell, quickly buffered by specific protein transporters and by sequestration in organelles such as mitochondria. Mitochondria play a key role in intracellular energy supply, especially in the neuron synapse regions, and are affected by ageing with changes in their structure and decay of their efficiency, leading to oxidative stress with increase in the cellular concentration of oxygen reactive species (for a review, see Toescu and Verkhratsky21). Age-dependent decay of the ionic exchange systems that normally induce rapid calcium elimination from the cytosol relates to various pathogenic pathways that may lead to apoptosis. The various biochemical mechanisms by which excessive calcium concentrations can be alleviated are good examples of the compensatory pathways that can take place in some cell populations to resist pathological ageing. However, it seems that specific neuron types are more exposed to the toxic effects of increased intracellular calcium concentration or the regulatory mechanisms elicited by this trend. For instance, hippocampal pyramidal cells are characterized by a decrease in the number of NMDA receptors with ageing; this effect may be a way to diminish toxic Ca2+ influx but also has the important consequence of a reduced efficiency of memory processes.22
Brain tissue depends vitally on a constant supply of oxygen and glucose by blood vessels. Ageing induces a progressive thickening and stiffening of arteries that alter the haemodynamics of cerebral vasculature and the efficiency of the transfer of energy metabolites to glial and neural cells. Hypertension and diabetes are major causes of such changes in the structure of blood vessels.23 The clinical significance of such vascular changes has already been emphasized above, concerning brain white matter and the periventricular lesions (hyperintensities in MRI) that are observed to increase with age, as a consequence of pathological changes in the small artery system in these deep brain regions (for a review, see Moorhouse and Rockwood24). In cohorts of very old patients, neuropathological findings have demonstrated an increasing prevalence of cerebrovascular lesions as a conjoint pathology of typical AD lesions.25
Changes in Large Neuroendocrine Systems and in General Behaviour During Ageing
Although not part of mainstream research, the influence of ageing on the whole neuroendocrine system is compelling. The most obvious example is the fall of ovarian estrogen production at menopause; estrogens, in addition to the cyclic interaction with its hypothalamic control pathways, are known to be involved in the control of mood, memory and cognitive and general behaviour.26 Akin to this pathway is the stress control system, mainly represented at the hypothalamic level by the corticotropin-releasing hormone (CRH) secreted by the paraventricular nucleus; this system, which undergoes inhibitory control from the hippocampus, is upregulated in depression and to a lesser extent in AD. The implication of the stress system in general behaviour is also compelling when considering that vasopressin secretion co-localizes with that of RCH. Vasopressin is also secreted in a rhythmic way by the supra-chiasmatic nucleus, which is viewed as the biological clock of the brain and is responsible for the circadian changes in the stress system.27 Ageing affects these neuroendocrine systems and the latter are involved in the major circadian behavioural changes, namely the sleep–wake rhythm (for a review, see Buckley and Schatzberg28
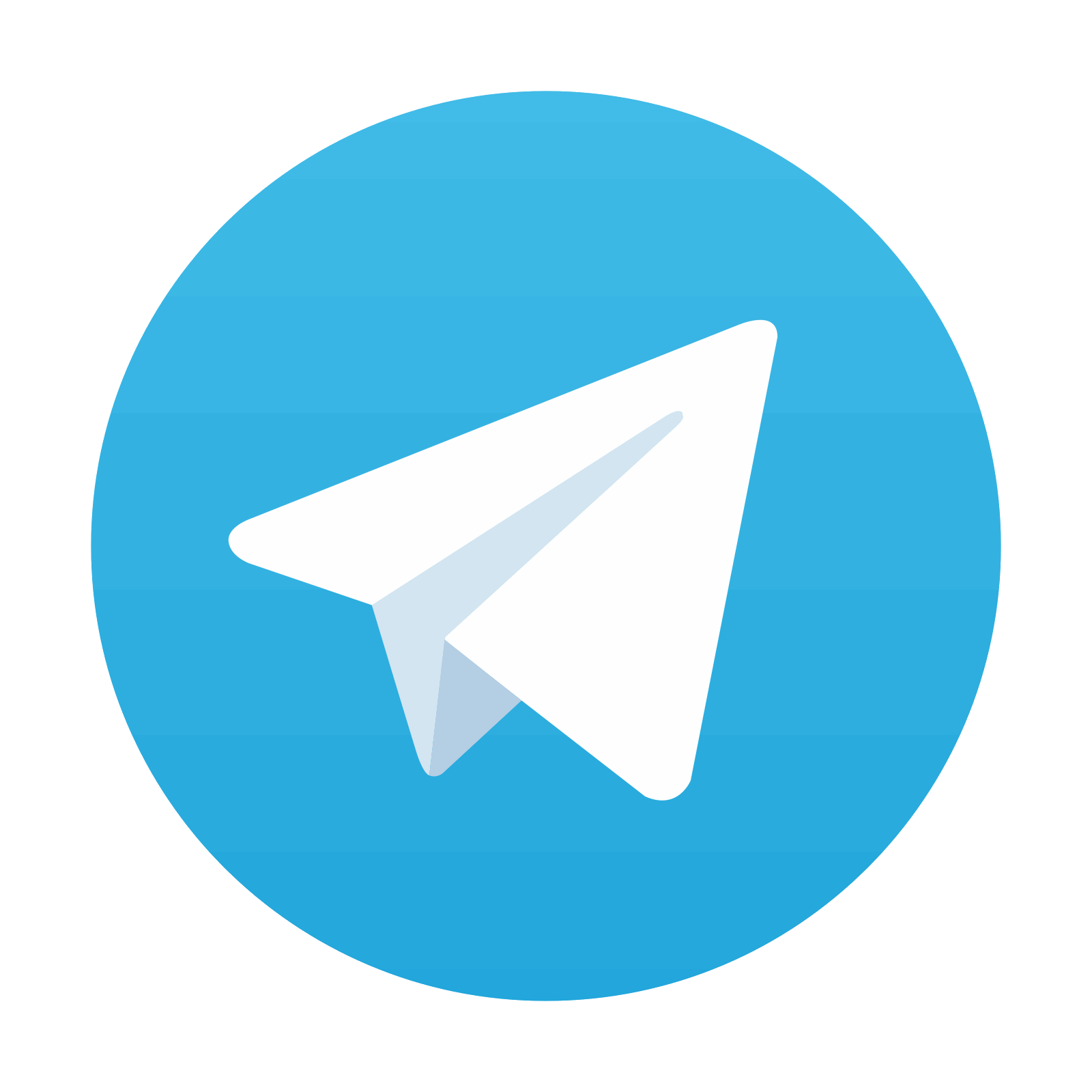
Stay updated, free articles. Join our Telegram channel

Full access? Get Clinical Tree
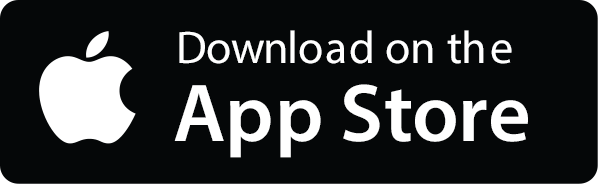
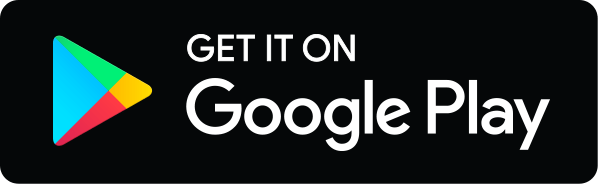