Aerospace Medicine Issues in Unique Aircraft Types
Richard S. Williams
Stephen L. Carpenter
Denise L. Baisden
Arnold A. Angelici Jr.
Stephen A. Bernstein
John A. Smyrski III
Dougal B. Watson
Shean E. Phelps
Kevin W. Williams
Melchor J. Antuñano
The greatest danger for most of us is not that our aim is too high and we miss it, but that it is too low and we reach it.
—Michelangelo
AEROBATICS
Unique Aspects of Aerobatics Flight
Aerobatic flight, like many advances in aviation, had its inception in warfare. Aircraft in World War I were initially employed in an observation role, but rapidly progressed to become powerful offensive weapons platforms. Pilots began shooting at each other, first with small arms and then with automatic weapons mounted on the aircraft. The ability to maneuver abruptly and swiftly to evade the enemy or gain a position of advantage became an absolute necessity, giving rise to the principles of aerial combat and, as an essential requirement for effective aerial maneuvering, aerobatic flight.
Aerobatic flight, according to the Federal Aviation Regulations, Section 91.303, is defined as an intentional maneuver involving an abrupt change in an aircraft’s attitude, an abnormal attitude, or abnormal acceleration, not necessary for normal flight. Aerobatic flight has long been recognized to have inherent risks, which, if not managed appropriately, can lead to catastrophic consequences for pilot, aircraft, and personnel on the ground. Some of these risks include failure of the airframe due to excessive acceleration forces, loss of situational awareness and increased chance of collision with other aircraft or the ground, and physiological compromise with loss of ability to control the aircraft. For these reasons, the Federal Aviation Administration (FAA) has placed restrictions against conducting aerobatic flight over congested areas, over an open-air assembly of persons, within airspace designated for an airport, within 4 nautical miles of the center of a federal airway, below an altitude of 1,500 ft above the surface, or when visibility is less than 3 statute miles. Aerobatic flight has great potential to reduce risk as well. Pilots who are trained in aerobatic flight are generally comfortable in most flight attitudes, including highly unusual ones. They more fully explore the entire flight envelope of aircraft, not just climbing, descending, and turning. They develop a better feel and sensitivity for aircraft. Aerobatic flying skills can be used to great effect in recovering from unusual flight attitudes that might occur as a consequence of severe turbulence such as that encountered in the wake of a large aircraft. Powerful vortices can be experienced when encountering wake turbulence, which can roll aircraft beyond 90 degrees of bank (even to inverted) and result in extreme pitch attitudes. Pilots trained in aerobatic flight have a greater chance of successfully recovering from such a situation without damaging the aircraft or impacting the ground. Many airline companies are sending their pilots to unusual attitude and aerobatic training, to enhance these skills and thereby reduce risk, and many general aviation pilots seek such training for similar reasons (1).
Aerobatic Maneuvers
Aerobatic maneuvers are essentially based on and permutations of the roll and the loop. A roll involves a continuous change in bank through 360 degrees generally about the longitudinal axis of the aircraft or at least about the direction of flight. A loop involves a continuous change in pitch, through 360 degrees, usually in a vertical direction. There are many variations of rolls and loops and in combination these constitute most of the aerobatic maneuvers commonly used and seen. Aerobatic maneuvers are characterized according
to G forces as well. “Inside” aerobatic maneuvers involve keeping the aircraft in a +Gz environment throughout the maneuver (see also Chapter 4). The traditional loop, begun from upright flight with a pull back on the control stick, is an example of an “inside” maneuver (Figure 27-1). “Outside” aerobatic maneuvers involve keeping the aircraft in a −Gz environment throughout the maneuver. The outside loop, begun from inverted flight with a push forward on the control stick, is an example of an “outside” maneuver (2) (Figure 27-2).
to G forces as well. “Inside” aerobatic maneuvers involve keeping the aircraft in a +Gz environment throughout the maneuver (see also Chapter 4). The traditional loop, begun from upright flight with a pull back on the control stick, is an example of an “inside” maneuver (Figure 27-1). “Outside” aerobatic maneuvers involve keeping the aircraft in a −Gz environment throughout the maneuver. The outside loop, begun from inverted flight with a push forward on the control stick, is an example of an “outside” maneuver (2) (Figure 27-2).
Types of rolls include the aileron roll (along the long axis of the aircraft, usually begins with a pitch-up) (Figure 27-3), the barrel roll (a simultaneous roll and pitch such that the aircraft flies through a helical pattern), the snap roll (an accelerated stall and horizontal spin), and the slow roll (roll while the aircraft remains in straight and level flight). A particularly beautiful rolling maneuver is the rolling 360 (the aircraft flies in a 360-degree horizontal turn while continuously rolling). Looping maneuvers include the (normal) circular loop and the square loop. Maneuvers that require looping and rolling include the avalanche (involving a snap roll, from inverted to inverted, at the top of a loop), the horizontal eight, the Cuban eight, the vertical eight, the cloverleaf (involving four loops in sequence with 90-degree vertical rolls separating the loops), the Immelmann (a half loop with a half roll to upright at the top of the loop), the split-S (a half roll to inverted flight followed by a half loop), and the vertical S.
Most of the looping aerobatic maneuvers, and some of the rolling maneuvers, can be performed as “inside” or “outside” maneuvers. Most pilots find outside maneuvers to be more technically challenging to perform, as well as being more uncomfortable due to the −Gz forces experienced (see also Chapter 4).
![]() FIGURE 27-1 The inside loop, involving continuous +Gz exposure. (Adapted from Robson DR. Skydancing: aerobatic flight techniques. Newcastle: Aviation Supplies & Academics, 2000.) |
It is important to note that “outside” maneuvers are almost the sole purview of civilian sport and competitive aerobatic flight. Military aerobatic flight, expressed as air combat maneuvering, occurs almost exclusively in the +Gz arena, with the exception of achieving transient zero G flight to increase airspeed. Few military pilots have chosen to exploit outside maneuvers, finding it quicker and more effective to remain in +Gz flight domains.
Civilian Aerobatics
Aerobatics are performed in the general aviation arena for purposes of pilot proficiency, unusual attitude training, recreation, competition, and for demonstrations and air shows. Aerobatic pursuits range from the weekend general aviation pilot performing limited maneuvers to highly skilled aerobatic pilots competing and performing in large air shows. The International Aerobatics Club, a division of the Experimental Aircraft Association (EAA) that advocates and fosters all aspects of aerobatics with an emphasis on safety, has developed categories of aerobatic pilots, based on skill levels, for purposes of competition. Competitive categories start with basic and proceed through sportsman, intermediate, advanced, and unlimited. Basic aerobatics include +Gz maneuvers such as upright spins, aileron rolls, and loops. Maneuvers become more complex as one goes through the categories, with −Gz maneuvers becoming
more prevalent in the advanced and unlimited categories. Aerobatic maneuvers, if performed properly and within the design specifications both of the maneuver and the aircraft, are not in and of themselves dangerous. However, if aerobatic maneuvers are performed improperly, if pilots are not appropriately trained and experienced, or if they have underlying physiological compromise, aerobatic maneuvers can result in significant risk. Overstressing an airframe or a pilot during aerobatics can and has resulted in catastrophic failure of either or both.
more prevalent in the advanced and unlimited categories. Aerobatic maneuvers, if performed properly and within the design specifications both of the maneuver and the aircraft, are not in and of themselves dangerous. However, if aerobatic maneuvers are performed improperly, if pilots are not appropriately trained and experienced, or if they have underlying physiological compromise, aerobatic maneuvers can result in significant risk. Overstressing an airframe or a pilot during aerobatics can and has resulted in catastrophic failure of either or both.
![]() FIGURE 27-2 The outside loop, involving continuous −Gz exposure. (Adapted from Robson DR. Skydancing:aerobatic flight techniques. Newcastle: Aviation Supplies & Academics, 2000.) |
The Physiological Challenges of Aerobatic Flight
Aerobatic flight involves rapid movement through all spatial planes in a controlled manner with resultant rapid changes in gravitational force vectors and in sensory input. This presents a very challenging environment with potential severe impact on neurologic, sensory, and cardiovascular systems (3). Gravitational forces are primarily in the Gz axis, with some Gx and Gy components in various maneuvers. For example, brisk linear acceleration experienced during an afterburner-assisted takeoff in a fighter aircraft or during a catapult shot from an aircraft carrier, induces +Gx, and snap rolls in general aviation aerobatic aircraft produce highly transient Gy exposures. The preponderance of force experienced in civilian aerobatic flight, however, remains both +Gz and −Gz. The detailed physiology of sustained acceleration is covered elsewhere in this textbook (see Chapter 4), so this chapter will concentrate on the specific gravitational forces and physiological effects experienced and reported by aerobatic pilots, especially in the civilian aerobatic sector.
The information presented in this chapter is, for the most part, based on data gathered at the 1996 National Aerobatic Championship competition at Fond-du-Lac, Wisconsin and at the 1996 Aerobatic World Championship competition in Oklahoma City, Oklahoma. Questionnaires as to the effects of aerobatic flight experienced were distributed and accelerometer readings were taken at the cockpits of competitive aircraft on landing immediately after competitive routines. The respondents were mostly advanced or unlimited aerobatic pilots.
Civilian aerobatic pilots generally wear parachutes, but employ no +Gz protective equipment during flight. Restraint systems are typically of the five-point type, including a −Gz strap, and some seat belts are equipped with rachet devices to further enhance stability in the seat. Some pilots reported participating in weight training and aerobic conditioning to assist their +Gz tolerance. Positive Gz protection includes muscle tensing and wide variations of the semiclosed glottisstraining maneuver, with no standardized training across the sport. There are no protective maneuvers for −Gz, but pilots reported that relaxation and adaptation with practice were helpful in minimizing untoward effects due to −Gz exposure. Gz levels experienced in civilian aerobatic flight are well into the ranges where untoward physiological effects may be seen. The Super Decathlon 8KCAB, an aerobatic aircraft designed primarily for training and +Gz maneuvers, has aerobatic load limits of +6 and −5 g. The Pitts S2-C aircraft, commonlyused for training, air shows, and advanced aerobatic competition, also has aerobatic load limits of +6 and −5 g. The Extra Aircraft 300L, designed for air shows and unlimited aerobatic competition, has flight load limits of +10 and −10 g, and the Sukhoi SU-31 has aerobatic load limits of +12 and −10 g.
Any of these aircraft are capable of achieving and sustaining Gz maneuvers that place pilots in an environment where all reported untoward +Gz effects. They are also capable of achieving extreme −Gz environments that represent hitherto largely unexplored flight regimes that will not be duplicated in any other aeronautical endeavors.
Positive Gz Effects
Advanced and unlimited aerobatic competitors and aerobatic air show pilots average peak +8 Gz exposures (peak +12 Gz recorded), with vertical turns producing the highest +Gz loads. Duration of exposures in these maneuvers range from 1.5 to 3 seconds, with turns executed rapidly and precisely. Pilots report a full range of +Gz effects, with more than 70% experiencing tunnel vision and gray-out (loss of peripheral vision), 30% reporting blackout (loss of vision), and 20% reporting at least one episode of G-induced loss of consciousness (G-LOC). G-LOC was experienced more commonly in aerobatic training aircraft, which are flown in lower Gz flight profiles (4-6 peak +Gz) but sustain the acceleration longer. Pilots agreed that the 3-second or less duration of exposure in advanced aerobatics was the primary protection against G-LOC. Other +Gz effects included skin rashes, back, neck and arm pain, headache, transiently decreased hearing, bright scotoma, muscle cramps, difficulty breathing, and urinary incontinence. Positive Gz tolerance increased with practice and repeated exposure.
Negative Gz Effects
Advanced and unlimited aerobatic pilots average peak −8Gz exposures as well (peak −10 Gz recorded), again with vertical turns producing the highest loads. Duration of exposures was similar to the +Gz regime. Approximately 60% of aerobatic pilots report headaches with −Gz exposure, especially early in the aerobatic season. Forty percent report bloodshot eyes, especially early in the aerobatic season, consistent with conjunctival petechial hemorrhaging. Red-out, a phenomenon that has never been adequately explained but may be due to gravitational effects on lower eyelids, was reported by 10% of pilots. Other −Gz effects included bright scotoma, red scotoma, eyelid rashes, eye pain, fatigue, and persistent vertigo in a few cases. This persistent vertigo, known as the wobblies amongst aerobatic pilots, is typically sudden in onset, can be near incapacitating in flight, and persists for days, weeks, or longer after onset. This entity, recently described as G-induced vestibular dysfunction (GVID), most closely resembles benign paroxysmal positional vertigo (BPPV) in its clinical expression (4). Its underlying pathology is most likely similar to that which occurs in BPPV, first described by Epley (5). Free-floating otoconia or cupular crystals are thought to migrate into the posterior semicircular canal, producing a false sense of angular motion with resulting vertigo and nystagmus with head movement in certain planes. Treatment with various modifications of the canalith repositioning procedures first described by Epley has been very successful in the treatment of BPPV (6). In GVID, liberation of otoconia and subsequent migration to the
posterior semicircular canal would be precipitated by shear forces induced by wide excursions in positive and −Gz exposure, accounting for the persistent vertigo characteristic of the disorder. Further research, including identification of cases of GVID and therapeutic trials of canalith repositioning, needs to be done.
posterior semicircular canal would be precipitated by shear forces induced by wide excursions in positive and −Gz exposure, accounting for the persistent vertigo characteristic of the disorder. Further research, including identification of cases of GVID and therapeutic trials of canalith repositioning, needs to be done.
The push-pull effect deserves special mention in the context of civilian aerobatics. Civilian aerobatic pilots have recognized since the early 1980s that +Gz tolerance is markedly reduced immediately following a −Gz exposure. Civilian aerobatic competitions have strongly discouraged or prohibited +Gz maneuvers immediately following −Gz exposures (without a short recovery period in the program) for over two decades, significantly antedating cognizance of this dangerous paradigm in the military and broader aeromedical communities (see also Chapter 4).
Fatigue, hydration status, and thermal stress are also important considerations and areas where risk can be increased in aerobatic flight if these stressors are not mitigated. G tolerance is reduced by all these factors. Most aerobatic aircraft have bubble-type canopies, and aerobatic competitions and air shows generally occur in the spring, summer, and autumn months. Temperatures in cockpits can be quite high, especially during ground operations and while waiting to takeoff. Adequate cockpit ventilation, hydration, rest, and conditioning are essential protective measures to observe in aerobatic operations.
Aerobatic Accidents
Every year there are accidents involving aerobatic aircraft, usually during practice or during air shows. Air shows pose the greatest risk because maneuvers are conducted at lower altitudes, at times only feet from the ground. Accidents generally involve loss of situational awareness and/or spatial orientation, with resultant collision with the ground or, in formation aerobatics, with another aircraft. Occasionally, airframe failures occur as well. Rarely, physiological compromise may be a contributing factor. The following accident report excerpts from the National Transportation Safety Board (NTSB) database typify aerobatic accidents.
A. NTSB Identification: IAD96FA126
On August 4, 1996, approximately 6.37 PM eastern daylight time, an Aerotek, Pitts Special S-1S, N2HT, was destroyed when a wing failed while performing aerobatics at the Three Rivers Regatta, and impacted in the Ohio River in Pittsburgh, Pennsylvania (7). The certificated commercial pilot/owner received fatal injuries. Visual meteorologic conditions prevailed and no flight plan had been filed for the local flight conducted under 14 Code of Federal Regulations (CFR) Part 91.
The pilot took off from Allegheny County Airport at 6.15 PM. After a period of holding, the pilot received clearance into the Regatta Aerobatic Box. A videotape taken of the accident showed the maneuvering by the pilot for the planned initial maneuver, a double snap roll. Within seconds of the beginning of the video, the lower left wing and a portion of the top wing failed. Both failed wings were seen folded aft against the empennage as the airplane descended and impacted the water inverted.
Witnesses interviewed after the accident and who knew the pilot stated that the pilot had told them that he used airspeeds higher than the manufacturer’s recommended maneuver airspeeds to obtain better performance from the airplane. Witnesses stated that they looked in the airplane on various occasions and saw higher positive and negative flight load factors than recommended by the manufacturer indicated on the load meter in the cockpit. “Witnesses acknowledged that the pilot voiced his concern to acquaintances about the airplane’s wings, and had purchased a set of wings for the airplane, because he had experienced flutter during recent flights.”
B. NTSB Identification: NYC07FA007
On October 14, 2006, approximately 1.00 PM eastern daylight time, an Extra Flugzeugbau Gmbh 300, N168EX, was destroyed when it impacted terrain at Culpeper Regional Airport (CJR), Culpeper, Virginia (7). The certificated commercial pilot was fatally injured. Visual meteorologic conditions prevailed, and the airplane was not operating on a flight plan. The local air show flight was being conducted under 14 CFR Part 91.
According to an FAA inspector who had been attending the show, the pilot had been performing aerobatic maneuvers for approximately 6 to 7 minutes along runway 04-22 when the accident occurred. At the time of the accident, the pilot was performing “multiple snap rolls on a 45-degree down line,” and during the maneuver, the inspector heard the announcer state that the airplane was in “the fourth turn of a five-turn demonstration.” The inspector also noted the altitude of the airplane in reference to the ground and shouted “NO,” as he “did not believe the aircraft could make another turn and clear the ground.”
A review of video footage revealed that the airplane completed two left-turning rolls at an approximately 45-degree descent angle, but during a third roll the trajectory changed toward a vertical descent. Following that, three additional left rolls were also completed in an approximately vertical-descent trajectory. After recovering from the last roll, the airplane stabilized in an estimated 45-degree nose-down, 20-degree left-wingdown attitude. The airplane continued to descend, and as a distant tree line came into the camera’s view, the airplane’s nose began rising, to where it was nose-level when the airplane impacted the ground. The airplane then disappeared into a depressed area behind the runway.
C. NTSB Identification: MIA98FA135A
A flight of four airplanes were participating in an air show. The flight leader of the demonstration team stated they had just completed an arrowhead formation (7). The number 2 and 3 airplanes moved into the diamond formation. The flight team completed the 5/8 loop portion of a 1/2 Cuban eight, set the 45-degree inverted downline, completed a
1/2 roll to the upright position, and was beginning the entry into a “clover” loop, when the accident occurred. Witnesses and video from TV stations indicated that the flight leader, number 3, and number 4 airplanes started pulling up out of a dive to initiate the diamond “clover” loop, when the number 2 airplane continued to descend and collided with the number 4 airplane. The NTSB determines the probable cause(s) of this accident as follows: the failure of the number 2 airplane pilot to maintain visual contact and/or proper position/clearance from other aircraft.
1/2 roll to the upright position, and was beginning the entry into a “clover” loop, when the accident occurred. Witnesses and video from TV stations indicated that the flight leader, number 3, and number 4 airplanes started pulling up out of a dive to initiate the diamond “clover” loop, when the number 2 airplane continued to descend and collided with the number 4 airplane. The NTSB determines the probable cause(s) of this accident as follows: the failure of the number 2 airplane pilot to maintain visual contact and/or proper position/clearance from other aircraft.
These NTSB reports all describe fatal accidents involving highly experienced civilian aerobatic air show pilots with many years of experience. There have also been catastrophic air show accidents involving military jet aircraft, some with massive fatalities on the ground as well, such as the Ramstein Air Show disaster of 1988, which caused more than 500 casualties among those in attendance (8). The failures to remain within human or aircraft performance limits or a moment’s lapse of concentration are commonly fatal errors in the unforgiving pursuit of air show aerobatics.
Civilian aerobatic air show pilots typically wear Nomex fire retardant gloves and flight suits to afford some protection in a potential postcrash environment. Some aerobatic competitors also use Nomex garments, but many do not. The use of helmets is rare in civilian aerobatics. An important recent innovation in civilian aerobatics is an ejection seat system designed and employed in Sukhoi aerobatic aircraft. The ejection system is pneumatically powered, involves immediate and automatic parachute deployment, and can ensure survival at speeds as low as 70 km/hr and altitudes as low as 15 m.
Aerobatic flight is thrilling to watch, exhilarating to perform, and enhances airmanship and pilot safety. Aerobatic flight can also subject pilots and aircraft to extreme gravitational exposures, spatial disorientation (SD), and loss of situational awareness, with potentially tragic results. The world of civilian aerobatics, with −Gz exposures that cannot be duplicated in any other sport or activity, certainly provides opportunity for interesting study of both short- and long-term physiological effects. Opportunities to interact with and study these unique pilots and their environment should be pursued by interested practitioners and researchers in the field of aerospace medicine.
AERIAL APPLICATION OPERATIONS
Unique Aspects of Aerial Application Flights
Aerial application is the delivery of substances, usually liquid chemicals, from the air to the surface of the Earth. The most common type of aerial application is the spraying of herbicides, pesticides, or fertilizers for agricultural purposes, commonly known as crop dusting. (Initially, most pesticides were applied in powder, or dust form, hence the term crop dusting. Now, most applications are liquid spray and aerial application is the preferred term.) Other forms of aerial application include the application of fire suppressants (“fire bombing”), mosquito control, and the application of herbicides for the purpose of eradicating plants used for illegal purposes (counterdrug activities).
From an aeromedical standpoint, this type of flying creates health concerns in several different areas. The low altitude of operations (sometimes only feet above the ground), rapid acceleration required during maneuvering, long duration of duty days, heat stress, and exposure to potentially dangerous chemicals are the primary hazards associated with this type of flying. Night application involves increased risks of obstacle contact. Secondary hazards include smoke exposure (fire fighting), lack of pilot redundancy, proficiency issues, and in the case of counterdrug operations the possibility of surface-to-air weapons discharge. Because these activities seldom involve the carriage of passengers and the operations usually take place in relatively unpopulated locations, the risk for casualty is limited primarily to aircrew. However, inadvertent misapplication of chemicals due to winds (overspray) or pilot miscalculation represents a potential hazard to collateral crops, livestock, or even humans.
The first recorded aerial application occurred in 1906 when John Chaytor used a tethered hot air balloon to seed an area of swampland in New Zealand. The first use of an airplane to perform aerial application occurred in 1921 when a modified Curtis Jenny was used to spread lead arsenate near Troy, Ohio to control sphinx moth caterpillars. In 1922, cotton fields in Louisiana were dusted to kill boll weevils. The first commercial crop dusting operation began in 1923 by a company that eventually became Delta Airlines. Aerial application of agricultural chemicals steadily became more common in the United States during the 1930s and 1940s, but the post-World War II period was the peak of activity as small family farms began to be amalgamated into much larger farming operations. It was during this time that aircraft were manufactured specifically for aerial application. In the late 1960s, however, environmental concerns resulted in increased regulation and a decline in the number of aerial applicators. Currently, there are somewhat more than 2,000 aerial application companies in the United States.
The first use of aerial fire-fighting in the United States occurred in 1930 when a Ford Tri-Motor aircraft dropped a load of water in a wooden beer keg. In 1955, a Boeing Stearman dropped six loads of free-flowing water on a fire in California.
Types of Aircraft
Almost any type of aircraft can be modified for aerial application. The most common types, however, are specifically designed single-seat fixed-wing aircraft, modified biplane aircraft, modified multiengine aircraft, and rotorcraft.
The advantages of a specifically designed aerial application aircraft include rugged construction for high-G
tolerance and superior crashworthiness, high power-to-weight ratio that allows better maneuverability and load capacity, high-lift wing design allowing lower-speed application, and better visibility for the pilot. The disadvantages include higher cost and lack of utility for nonaerial application activities.
tolerance and superior crashworthiness, high power-to-weight ratio that allows better maneuverability and load capacity, high-lift wing design allowing lower-speed application, and better visibility for the pilot. The disadvantages include higher cost and lack of utility for nonaerial application activities.
The advantages of a modified biplane include the relatively lower cost, high-lift wings, good visibility, and some utility in nonaerial application activities. The disadvantages include the need to modify the aircraft, an open cockpit that could increase the pilot’s exposure to the chemical being applied, and decreased crashworthiness.
The modified multiengine aircraft (used for aerial fire fighting) has a vastly superior delivery capacity, greater utility in nonaerial application activities and usually has the advantage of multiple crewmembers. The primary disadvantages are the significantly increased cost (both of acquisition and operation) and generally higher operational altitudes required by its decreased maneuverability.
Rotorcraft provides exceptional maneuverability and outstanding visibility, but this is counterbalanced by a somewhat diminished delivery capacity and relatively high cost.
Training Issues
Aerial applicators are required to hold a commercial pilot certificate and have additional training in aerial application. This additional training is acquired through schools specializing in agricultural aviation and augmented by on-the-job training with experienced aerial applicators. The aerial applicator must also be trained for and successfully obtain a Commercial Pesticide Applicators license.
Low-Altitude Activities
Most aerial application takes place as close to the ground as possible. This insures maximum delivery of chemical to the intended site with minimum overspray (the application of chemicals to unintended sites). Obviously hypobaric medical problems are usually not a concern; however, the risk of contact with obstructions is significantly increased in low-altitude operations. The pilot’s attention during these operations must be split between the need to assure that the aerial application is proceeding properly and the need to be vigilant for obstructions. This creates a high task load that requires the pilot to be at peak performance for an extended period of time. As a pilot reaches the end of a pass over the delivery site, he must discontinue the application and rapidly gain altitude to avoid obstructions that might include fences, roads, buildings, power lines, or rising terrain. If the operation requires multiple passes, he must then turn the aircraft as efficiently as possible, rapidly return to, and level off at delivery altitude, while avoiding the obstructions and reactivating the application. All of these activities must take place in a matter of seconds.
Clearly, any medical condition that impairs a pilot’s ability to make rapid judgments and react instantly has the potential to be catastrophic. Although sudden medical incapacitation is always a small risk, fatigue, distractions, medications, and alcohol are much more likely to affect the pilot’s ability to perform at low altitude. It is critical that the pilot assess himself before and during each flight for any suggestion that he is not at the “top of his game” and then adjust his operations accordingly.
Acceleration Exposure
The efficient application of spray requires rapid changes in aircraft direction and altitude over short geographic distances. The result is exposure to high G loads, both by the aircraft and the pilot. It is routine for crop dusters to pull 2 to 4 Gs dozens of time daily in low-altitude situations in close proximity to obstructions. Most exposures are +Gz. (see also Chapter 4). Although this level of G exposure is unlikely to impair trained healthy individuals, it can be more problematic under certain circumstances. Clearly repeated G exposure leads to fatigue that can then diminish G tolerance. The so-called push-pull phenomenon, such as might occur when an airman pushes over an obstruction and then pulls up close to the ground to begin a spraying pass, can also decrease G (see also Chapter 4). Aviators who are under treatment for hypertension can be subject to decreased G tolerance from medication effects. Overexposure to chemicals used for spraying, especially organophosphates, can impair the sympathetic nervous system to the point of diminished G tolerance. In all these circumstances, the effect on G tolerance can lead to reduced cerebral perfusion with accompanying impairment of cognitive and visual ability. This is likely to be more problematic as dusk approaches late in the workday.
Prolonged Crew Duty Day
Most aerial application operations occur in time-critical situations. Obviously in fire fighting operations, the more retardant that can be delivered, the quicker the fire can be contained. This may mean long days of flying as many missions as possible to maximize the delivery of retardant. Similarly, crop dusting must be accomplished during a relatively small “time window of opportunity” when the growing conditions are right for the delivery of chemicals. A busy crop dusting service will have many fields over a wide geographic area to spray during this period of time, so sunrise to sunset operations 7 days a week is the rule. Clearly, a series of long duty days leads to accumulating fatigue and could potentially increase the risk for inattention to tasks.
Heat Stress
With the exception of certain fire fighting and night operations, aerial application takes place during daylight hours, mostly during the spring and summer growing seasons when it is not raining. This means that the pilot is exposed to warm weather and direct sunlight. When this is combined with low-altitude operations and slow flight, which limits the flow of cooling air through the cockpit, there is a significant risk for heat stress (see also Chapter 7). The use of air-conditioning in some aircraft helps counter this risk, but many crop dusting aircraft do not have this
option available. It is critical that aircrew stay well hydrated during warm weather operations. Unfortunately, some pilots are reluctant to drink extra fluids because that leads to the need to urinate that often requires shutting down the operations.
option available. It is critical that aircrew stay well hydrated during warm weather operations. Unfortunately, some pilots are reluctant to drink extra fluids because that leads to the need to urinate that often requires shutting down the operations.
Exposure to Chemicals
The most potentially hazardous chemicals used in agricultural aerial application are organophosphorus compounds. Organophosphates are pesticides that work by irreversibly blocking acetyl cholinesterase. The result is unabated cholinergic neuromuscular activity. This is the desired effect in agricultural pests, but humans are subject to the same toxicity with a sufficiently acute exposure. Indeed, some of the most common military nerve agents are organophosphates. Aerial applicators are exposed to these substances when they are mixed and loaded, while they are being delivered, and when the aircraft is cleaned up after spraying. Symptoms of acute poisoning include diaphoresis, lacrimation, salivation, gastrointestinal cramping, and bronchospasm. Left untreated, central nervous system stimulation with seizures and respiratory failure can develop. A recent study (9) has suggested a significant correlation between chronic low-level organophosphate exposure and chronic neurologic symptoms, including fatigue, depression, absentmindedness, and difficulty concentrating. Clearly, these are symptoms that cannot be tolerated in aerial applicators. There was a lower correlation of these findings with other pesticides, herbicides, and fungicides.
Most Common Contributing and Casual Factors in Accidents
As one might expect, aerial application accidents are much more likely to occur during the application-related operation itself, rather than on takeoff or landing. In fact, 62% of aerial application accidents occur during the maneuvering part of the flight compared with 9% for general aviation accidents (10). Because most of this flying is at relatively low speeds and altitudes, crash survival is easily possible with good aircraft design. Modern aerial application aircraft incorporate several features to increase survivability, including roll cages, five-point restraint systems, crushable fuselage components (including floor and landing gear), engine mount design that pushes the engine down and under the cockpit in a head-on collision and a lack of protruding, noncollapsible objects in the cockpit.
LIGHTER-THAN-AIR OPERATIONS
The first lighter-than-air (LTA) vehicle dates back to the Montgolfier brothers whose hot air balloon first successfully carried Jean-Francois Pilatre de Rozier and Marquis Froncois d’Arlandes on November 21, 1783 in Paris, France. That flight was quickly followed by the flight of Jacques Charles who flew in a balloon filled with LTA hydrogen gas. In 1785, de Rozier flew a third type of balloon, a combination hot air and hydrogen balloon. It was designed with a hydrogen envelope inside a hot air envelope. Unfortunately, the combination of highly flammable hydrogen gas with an ignition source resulted in an explosion that killed both de Rozier and his passenger. The Roziere combination balloon was ignored until nonflammable helium gas became more easily available (see also Chapter 1).
Balloons have served a variety of purposes over time. Balloons were used as battlefield observation platforms reporting enemy troop positions and directing the artillery fire as early as the French Revolution. Zeppelin airships were used by the Germans in World War I to make occasional bombing raids on targets in Great Britain and to transport heavy loads over long distances. Airships were used during World War II for search and rescue, photographic reconnaissance, convoy escort, and antisubmarine patrols. Balloons have also been used to gather scientific information on the upper atmosphere. Currently ballooning is primarily a sport activity in the United States. Airships are used commercially primarily for advertising or television photography of sporting events. They are also used in border patrols and fire observation (11, 12, 13, 14).
Types of Lighter-than-Air Vehicles
There are several types of LTA vehicles: hot air balloons, cold air or gas balloons, combination balloons, and airships. These are termed lighter-than-air because they are buoyant. How that buoyancy is achieved depends on the type of vehicle. Balloons consist of a bag, called the envelope, from which a basket, or gondola, is suspended. The gondola carries the heat source that is usually fueled by propane. In hot air balloons, buoyancy is achieved by heating air in the balloon envelope allowing the gas to expand and become less dense. The less dense air is LTA outside the balloon and the balloon therefore rises.
Cold air balloons have the same components as hot air balloons except for the lack of a heat source. The envelope, however, is sealed at the bottom. In cold air balloons, buoyancy is achieved by filling the balloon envelope with a type of gas that is LTA, thereby causing the balloon to rise. Hydrogen, helium, and ammonia have been used as lifting gases. When helium became more easily available, interest increased in the Roziere combination balloon. Currently, Roziere balloons use a helium inner envelope surrounded by a hot air envelope heated by propane. This type of balloon was used by Steve Fossett to fly solo around the world in 2002 (15).
There are three classes of airship—rigid, semirigid, and nonrigid. The current airships, or blimps, are typically of the nonrigid type, also called pressure airships. These airships have a large envelope that contains the lifting gas. Air-filled bags, called ballonets, are located inside the envelope, and act as ballast regulating the internal pressure. The ballonets deflate or inflate with air as the helium expands on ascent and contracts on descent. The airship also has a gondola for carrying the passengers, crew, and cargo with gasoline-powered engines on either side. The flight control surfaces
(rudder, fins, and elevators) provide stability and the ability to steer the vehicle.
(rudder, fins, and elevators) provide stability and the ability to steer the vehicle.
Semirigid airships, like the new Zeppelin NT (Neue Technologie) airships, are pressure airships that have a lower rigid keel structure. The rigid airships, like the old Zeppelins, initially had a metal framework containing a gas-filled bag. The Hindenburg fire, however, essentially ended the use of the rigid airship.
Special Nature of Lighter-than-Air Flying
In hot air balloons, lift is controlled by changing the temperature of the air inside the envelope. To ascend, the air is heated more. To descend, the air in the balloon is allowed to cool naturally for a slow descent, or air is released through a parachute vent at the top of the balloon for a more rapid descent.
Directional control in a balloon is very limited—the balloon is dependent on the direction of the wind. Because wind direction and speed varies depending on the altitude, the balloon pilot can change the direction of the balloon by changing altitude. One of the difficulties with flying a balloon is dealing with the delayed response in slowing or stopping a descent. Stopping a descent can take more than 30 seconds from the time the burner is lit until the descent stops. The pilot, therefore, needs to plan carefully.
Airships have more directional control because they are powered by engines and have flight control surfaces. On takeoff, the airship pilot vents “heavy” air from the ballonets to make the airship positively buoyant. The pilot will also use the engine and elevators to angle the blimp into the wind to provide additional lift. By filling the ballonets with air and adjusting the elevators, the airship pilot descends. This again requires careful planning on the part of the pilot to avoid being “too light” on landing.
Environmental Exposure Considerations
Balloons and airships typically operate at relatively low altitudes. Hypoxia and trapped gas problems are rarely an issue. Heat or cold exposure may, however, be an issue and appropriate clothing for the weather should be worn. Windchill is not typically a factor because the balloon moves with the wind.
Ballooning is very weather dependent and typically requires clear skies and calm winds. Storms, with the possibility of lightning strikes, are extremely dangerous for balloons. Rain can also decrease visibility and even damage the balloon envelope. Balloons typically fly early in the morning just after sunrise, or late in the day just before sunset, to ensure winds are calmest.
Airships can fly in more weather conditions than balloons. With its slow speed and responsive controls, the airship can typically handle turbulence better than an airplane. Icing is also less of an issue than with an airplane. Airships are capable of flying in all the weather conditions that a fixed-wing aircraft can; however, airships must avoid takeoff or landing in wind speeds greater than 30 knots.
Personal Protective Equipment
Some personal protective equipment is recommended for balloon pilots. To protect the balloon pilot from burns in dealing with the heat source, flame-resistant gloves should be worn such as leather or nomex gloves. The pilot’s clothing should include long sleeves and long pants and be made of nomex or natural fibers that will not burn readily. In addition, the pilot should wear eye protection (may simply be sunglasses) to shield the eyes from heat should a gust of wind blow flames toward him or her. Balloon systems that hang the burner from the envelope instead of supporting it in the basket would require helmets be worn by the pilot and passengers. The ground crew also should wear gloves whenever handling the ropes or lines to prevent rope burns.
Accident Data
A review of the NTSB accident database for balloon accidents from 2005 to 2006 revealed 20 accidents involving 92 persons (16). Sixty-three of the 92 individuals were uninjured, 10 suffered minor injuries, 16 suffered serious injuries, and 3 were fatally injured. Two of the fatalities were due to the basket being engulfed in flames after collision with power lines. The other fatality was due to collision with boulders on top of a hill. Although the injuries were not always specified in the report, most serious injuries involved fractures (arms, ankle, pelvis, vertebrae, and clavicle). Two of the serious injuries involved ground crewmembers. In one case, the ground crewmember became entangled in the balloon vent lines and was lifted off the ground as the balloon ascended to approximately 75 ft. The pilot was unable to lose altitude because of the entangled vent lines. While attempting to get the ground crewmember close to a tree, the balloon struck the tree; the crewmember became untangled and fell through a barn roof. In the other case, the ground crewmember grabbed onto the basket of the balloon as it landed. The ground crewmember slipped on wet grass and continued to hold onto the basket as the balloon lifted into the air again. When the balloon reached approximately 8 ft in the air, the grip on the rope was lost and the crewmember impacted the ground.
Human error was listed as a probable cause of the accidents in 70% of the cases. Wind (high winds, wind gusts, downdrafts, and wind shear) was listed as a cause or contributing factor in 11 of the 20 accidents (55%). Fourteen of the 20 accidents (70%) occurred during landing with 10 of those resulting in serious injuries. This is consistent with other studies of balloon accidents. De Voogt and van Doorn reviewed balloon accident data from 2000 to 2004 (16). During this 5-year period, 86 accidents occurred with 85% of the accidents occurring during landing procedures. Fifty-eight percent of those involved in the accidents were uninjured, 23% suffered minor injuries, 18% were seriously injured, and 1% were killed. A review of hot air balloon accidents in the United Kingdom from 1976 to 2004 again revealed that most accidents occurred during the approach and landing phase of flight with adverse weather present in a number of the accidents (17).
A review of the NTSB accident database for airship/blimp accidents from 2002 to 2006 revealed three accidents and one incident involving a total of seven persons (18). Six of the seven individuals were uninjured and one suffered minor injuries. There were no serious injuries or fatalities. Seventy-five percent of the accidents/incidents were caused by human error. Weather and winds were also a factor in three of the four accidents/incidents. Similar to the balloon data, the majority (75%) of the airship accidents/incidents involved the landing phase of flight. In one accident, the pilot encountered weather and downdrafts, lost control of the airship and collided with trees and transmission wires. In another accident, the airship was “very light” during the landing. The accident occurred as the pilot attempted to abort the third landing attempt and impacted a fence and a lumber pile. The one incident occurred as the crew was securing the airship after landing. A wind gust blew the airship into nearby trees. The remaining accident occurred during the takeoff phase of the flight. A wind gust blew the ship to the right. The ground crew manning the nose ropes could not control the movement. The pilot attempted takeoff, but the landing gear hit a fence and then the airship settled onto a one-story building. A second wind gust pushed the airship into the next building over.
Crashworthiness
The design of the hot air balloon with its wicker basket gondola provides little in the way of crash protection. The limited directional control in a balloon also makes crash avoidance more challenging. Despite these issues, the accident data shows that the vast majority of individuals involved in balloon accidents are either uninjured or suffer only minor injuries.
The airship’s construction and control systems make it very safe to operate. All of the individuals involved in the airship accidents discussed previously were either uninjured or suffered minor injuries. Because the pressure inside the airship envelope is very low (˜1/15 psi), a small hole in the envelope would cause the gas to escape very slowly taking hours or even days to affect the airship’s performance. A large hole in the envelope would likely abort the mission, but would still allow the airship plenty of time to return to its base for the needed repairs.
LTA operations offer a unique aspect of aviation with slow speeds and quiet operations. LTA pilots are not required to hold a medical certificate. Despite having little medical oversight, however, preexisting medical issues were not found to contribute to any of the accidents reviewed.
ULTRALIGHT OPERATIONS
Ultralight aircraft are defined by the Federal Regulations under Part 103—Ultralight Vehicles (19). These vehicles are, except for the purpose of instruction, intended to be manned by a single occupant, used for sport or recreational purposes, weigh less than 155 lb (70.3 kg) if unpowered and less than 254 lb (115.3 kg) if powered. If the vehicle is powered, it is not to have a fuel capacity of more than 5 gal. These weight limits do not include floats or safety devices, such as a ballistic parachute, which are intended to be deployed in a potentially catastrophic situation. Ultralight vehicles are also limited to a full power speed in level flight of not more than 55 knots (101 km/hr) calibrated airspeed and a power off stall speed that is not to exceed 24 knots (44 km/hr) calibrated airspeed. Ultralight vehicles and their component parts are not required to meet the same airworthiness certification standards specified for aircraft or to have certificates of airworthiness (20).
Types of Ultralight Vehicles
Ultralight operations include aircraft in the following categories: glider or sailplane, motor gliders, paragliders (Figure 27-4), motorized paragliders, hang gliders, balloons, and ultralights.
Limitations to Operate Ultralight Vehicles
The operators of ultralight vehicles are not required to meet any aeronautical knowledge, age or experience requirements, or to have a medical certificate to operate this class of vehicles (20). Although the operators of ultralight vehicles are not required to hold a valid medical certificate to operate this class of vehicles, they must still comply with the part of the CFR that cover the certification of pilots, flight instructors and ground instructors, specifically, Part 61.53 Prohibition of operations during medical deficiency (21). CFR Part 61.53 states that a person may not act as pilot in command while that person is known to have a medical condition that would make the person unable to operate the aircraft in a safe manner (21). The operator of an ultralight vehicle, who does not possess a valid medical certificate but possesses a valid U.S. driver’s license, must comply with each restriction and limitation imposed by that person’s U.S. driver’s license and any judicial or administrative order applying to the operation of a motor vehicle (22). Operators are not subject
to the restriction that applies to sport pilots; the ultralight operators do not have to be eligible for a medical certificate. CFR Part 103.7(b) Certification and Registration does not state that if they have been denied a medical certificate they cannot operate their vehicles. Nothing is mentioned in CFR Part 103 about restrictions if they were previously denied a medical certificate, nor is anything mentioned about requiring the operator to have a valid U.S. driver’s license, as long as they comply with Parts 61.23(b) 61.53(b), and they are able to operate the vehicle in a safe manner.
to the restriction that applies to sport pilots; the ultralight operators do not have to be eligible for a medical certificate. CFR Part 103.7(b) Certification and Registration does not state that if they have been denied a medical certificate they cannot operate their vehicles. Nothing is mentioned in CFR Part 103 about restrictions if they were previously denied a medical certificate, nor is anything mentioned about requiring the operator to have a valid U.S. driver’s license, as long as they comply with Parts 61.23(b) 61.53(b), and they are able to operate the vehicle in a safe manner.
![]() FIGURE 27-4 Training paraglider making a pass over Torrey Pines California. Photo courtesy of Dr. Arnold A. Angelici, Jr. |
Other limitations to the operation of ultralight vehicles include the hours of operation and where they can be operated. Ultralight vehicle operations are restricted to the time between sunrise and sunset. If the vehicle is equipped with an appropriate anticollision lights, the time of operation can be extended to 30 minutes before sunrise and up to 30 minutes after sunset. All ultralight vehicle operations are restricted to uncontrolled airspace and are not to be operated over congested areas of a city, town, or settlement (23,24). The flight visibility in uncontrolled airspace, at or below an altitude of 1,200 ft above the ground level (AGL), must be 1 statute mile and clear of clouds. If the ultralight vehicle is flying more than 1,200 ft AGL, the visibility must be 5 statute miles or greater (25). Operators of ultralight vehicles are also expected to follow the same general operating rules and regulations governing all other aircraft. These include operations in controlled airspace and, restricted airspace, flight restrictions in the proximity of areas designated by notice to airmen (NOTAM) (25).
Personal Protective Equipment
The type and amount of personal protective equipment will vary depending on the vehicle flown. Open structure vehicles, such as paragliders, motorized paragliders, or hang gliders would require, for safe operation, that the operator wear helmet and goggles for head and eye protection. Clothing should cover the arms and legs, along with gloves and shoes, to provide protection from windblast, temperature extremes, and airborne particles such as insects or debris carried aloft by thermals or other weather phenomena. The clothing may be made of fire-retardant material if the vehicle is powered; the possibility of fire exists because of fuel carried and a source of ignition is present. Safety belts, four- or five-point type seat belts for upright seated vehicles, or a harness for prone, “weight shifting controlled” vehicles, should be installed and used with the intention of protecting the operator from injury due to sudden acceleration or deceleration forces that were or were not intended to be experienced by the operator. Parachutes have been recommended to be worn in all sailplanes and gliders (Figure 27-5). There are two types of parachutes, the type worn by the operator of the vehicle and the other is an airframe parachute. The airframe parachute system, introduced in the early 1980s, is attached to the ultralight’s airframe. If there is a need to utilize the parachute because of structural failure or unrecoverable loss of control, the parachute is deployed and the operator remains with the ultralight vehicle throughout the recovery. Some of these systems are referred to as ballistic recovery systems because the parachute is deployed using an explosive or a compressed air charge (26). The structure surrounding the operator of the vehicle is also part of the crash protection and is intended to absorb some of the impact forces experienced on landing with the parachute.
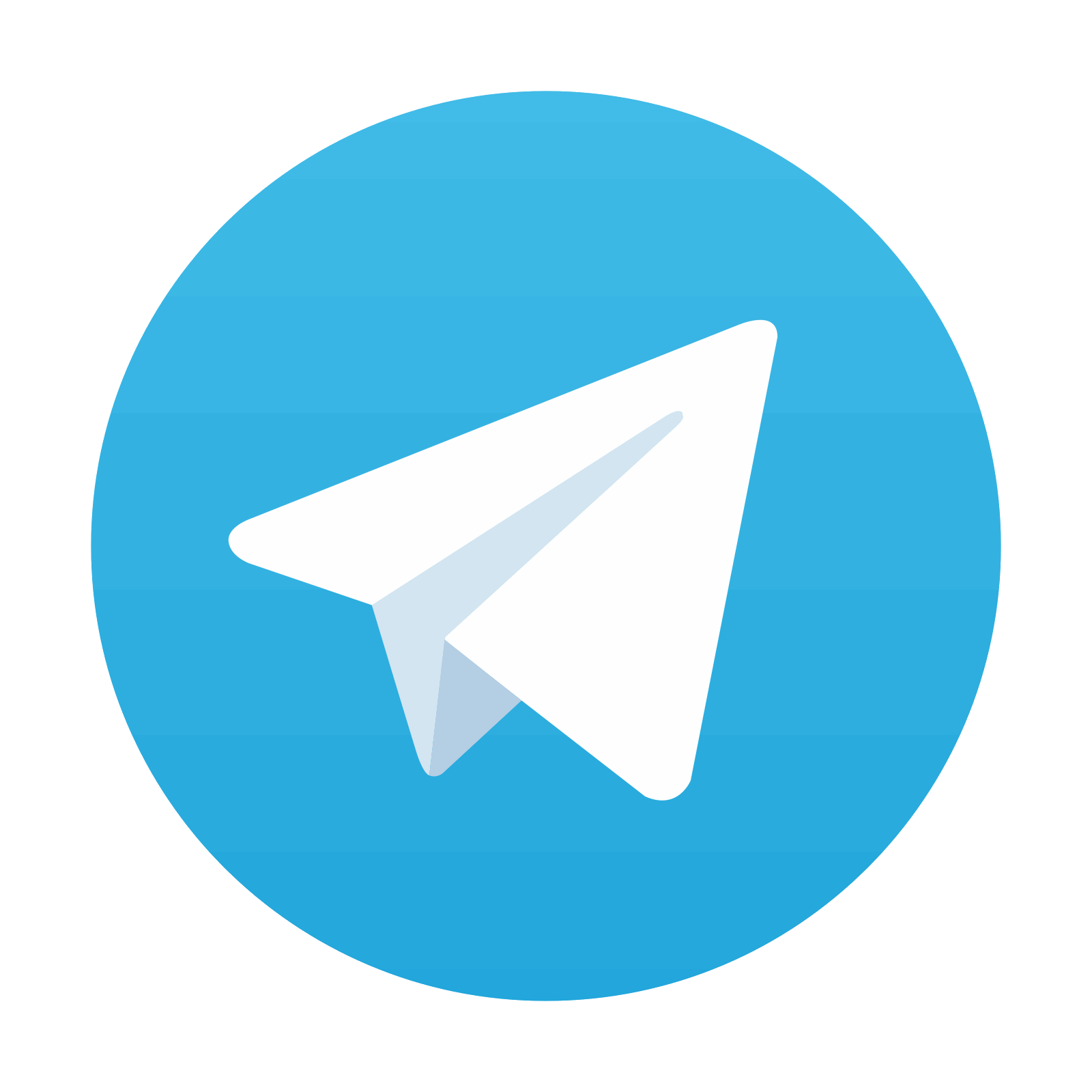
Stay updated, free articles. Join our Telegram channel

Full access? Get Clinical Tree
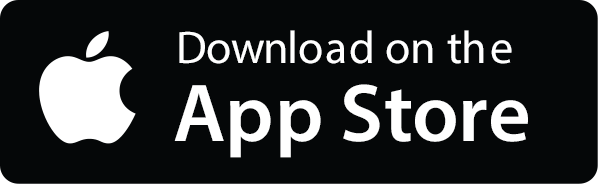
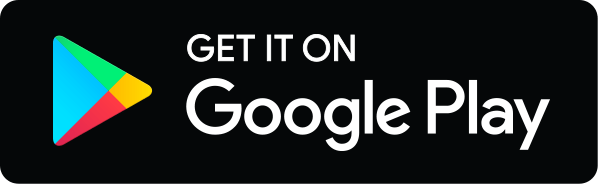