Introduction
Diabetes is one of the fastest-growing health challenges of the 21st century, with the number of adults living with diabetes having more than tripled over the past 20 years . The International Diabetes Federation reported that in 2019, the prevalence of diabetes was 9.3% (463 million people worldwide), with a predicted rise to 10.9% (700 million people) by 2045 . Furthermore, it has been shown that over 1.1 million children and adolescents below 20 years have type 1 diabetes. On top of these staggering figures are the number of people with impaired glucose tolerance (IGT) or metabolic syndrome at 373.9 million in 2019 (7.5%) and a predicted rise to 548.4 million (8.6%) by 2045 .
There were 4.8 million people with diabetes in the United Kingdom alone in 2019, and this is rising. Figures from Diabetes UK shows that someone is diagnosed with diabetes every two minutes, with 5.3 million expected to be living with the condition by 2025 .
Diabetes is strongly associated with both micro- and macrovascular complications. As a result, 10% of global health expenditure, equal to USD 760 billion, is directed toward diabetes and its complications . Microvascular changes lead to nephropathy, retinopathy and neuropathy. Among these diabetes-associated complications, diabetic peripheral neuropathy (DPN) is the most common and costly, occurring in around 50% of individuals with diabetes . Distal symmetric polyneuropathy (DSPN) typically follows a distal-proximal course and results in symmetrical symptoms and signs between the body’s left and right sides. Common symptoms include burning, numbness, tingling, pain, and/or weakness starting in the distal lower extremities, which progress into more extreme symptoms of neuropathic pain in around 10%–30% of affected patients . Symptoms may be sporadic or constant but can be debilitating and in many people lead to depression, sleep disorders and overall reduced quality of life .
The true prevalence of DPN is underestimated as its assessment is challenging. However, DPN is recognized as the most common complication of diabetes.
DPN is the strongest initiating risk factor for diabetic foot ulceration (neuropathic ulcer) , and existing ulcers may be further exacerbated from damage to sensory neurons. Resultant limb numbness causes ulcers to remain undetected for longer periods ; thus corrective actions are not taken nor advice sought at the early stages of the disease. Often the first sign that a person has DPN is a foot ulcer, which may lead to irreversible tissue damage, lower limb amputation, and significant morbidity.
People with diabetes account for more than 40% of hospitalizations for major amputations and 73% of emergency admissions for minor amputations in the United Kingdom. A single diabetes-related foot ulcer can take over 240 days to put into remission and costs £8,000 per annum (pa) to treat. Ulcers frequently recur and eventually may require the amputation of a lower limb. DPN is hugely costly to our NHS (>£1.1 billion pa in direct medical costs) and the wider UK economy (~£4 billion) and is particularly debilitating and distressing for patients and their families. It can also lead to untimely death , with 5-year mortality ranging from 52% to 80% after major amputation .
Furthermore, with diabetes-related lower limb amputations increasing at the rate of almost 20% pa in line with the increasing prevalence of diabetes, there is a huge strain on NHS budgets that are unable to keep up.
Autonomic neuropathies are a class of DPN that share similar diffuse pathophysiology with DSPN but differ by being largely nonsensory . These typically affect the cardiovascular, urogenital, and gastrointestinal systems. Patients may also suffer from sudomotor dysfunction, hypoglycemia obliviousness and abnormal pupillary function . Rare forms of DPN include mononeuropathies, polyradiculopathies, and treatment-induced neuropathies . These atypical forms are generally self-limiting and resolve with medical management and physical therapy, usually over several months .
In clinical settings, there are several different approaches to assess DPN, and the choice of the test will depend on the aim of testing. It is usually sufficient in a busy clinic to establish whether a patient is symptomatic, particularly of painful DPN , and whether or not they are at high risk of foot ulceration, typically through monofilament testing. However, to fully assess the damage and phenotype of DPN, sensory deficits must be detected early. Those accurate biomarkers are available for monitoring of DPN and for use in clinical trials of potential new treatments.
Currently, there are no simple markers for early detection of DPN in routine clinical practice. The measures we use are crude and detect the disease very late in its natural history. Even the benefits gained by standardizing clinical assessment with scored clinical assessments remain subjective being heavily reliant on the examiners’ interpretations.
In this chapter, we review the current knowledge and the optimal approaches for diagnosis and screening of DPN.
Types of nerve fibers
Peripheral nerve fibers can be classified using Erlanger and Gasser’s classification, which defines nerves based on diameter, conduction speed, and myelination level ( Table 4.1 ). A-fibers have the largest diameter, with the thickest myelination and fastest conduction speed, and act as sensory and motor fibers within the somatic nervous system. They may be further divided into large nerve fibers that have sensory and motor functions (Aα and Aβ), and small nerve fibers (Aγ which has motor functions, and Aδ, which may be autonomic or sensory fibers) .
Classification | Myelination | Diameter (um) | Conduction velocity (m/s) | Type | Function |
---|---|---|---|---|---|
Aα (alpha) | Yes | 12–22 | 70–120 | Sensory/motor | Proprioception, touch sensory, somatic motor to extrafusal muscles |
Aβ (beta) | Yes | 5–12 | 30–70 | Sensory/motor | Proprioception, touch/pressure sensory, somatic motor to intrafusal muscles |
Aδ (delta) | Yes | 1–5 | 5–30 | Sensory | Touch and cold thermoreceptors, nociception |
Aγ (gamma) | Yes | 2–8 | 15–30 | Motor | Somatic motor to intrafusal muscles |
B | Yes | <3 | 3–15 | Autonomic | Visceral afferent fibers and preganglionic efferent fibers |
C | No | 0.1–1.3 | 0.6–2 | Sensory/autonomic | Temperature (warm receptors), pain perception, nociception, itching |
Group B-fibers are small, with moderate myelination and slower conduction velocities than A-fibers. B-fibers act mainly as general visceral afferent and pre-ganglionic fibers and are found only in the autonomic nervous system.
Group C-fibers have a small diameter, low conduction velocity, and are the only unmyelinated group. They act as somatic, afferent fibers that carry sensory information relating to temperature and pain and have autonomic functions such as the stimulation of the sweat glands .
Screening
The American Diabetes Association (ADA) and the International Working Group on the Diabetic Foot (IWGDF) recommend regular examination of people with DM for the diagnosis of DPN and loss of protective sensation using simple standard tests for the identification of those at risk for diabetic foot ulcer . It is recommended that all patients with type 2 diabetes mellitus (T2DM) be screened for DPN at diagnosis, and for type 1 diabetes mellitus (T1DM), the screening should begin 5 years postdiagnosis . After this initial screening, all patients should be reviewed annually.
Nerve conduction studies (NCS) are considered the gold standard for the diagnosis of large-fiber neuropathy. The Toronto consensus recommended the use of abnormal NCS with a symptom or sign to diagnose DPN. However, the need for specialist examiners and equipment renders NCS inappropriate as a screening test. Thus it is used only to confirm any possible/probable DN picked up postscreening using other measures .
More commonly, screening for DPN involves history taking for neuropathic symptoms and examination of the feet, along with a screening test . Traditional screening tests benefit from being quick and easy; however, such as NCS, these only assess large-fiber function and are unable to detect any early changes in small nerve fibers. Furthermore, two systematic reviews focusing on the use of monofilament testing, a commonly used screening test for DPN ( Fig. 4.1D ), described a variation of diagnostic value in the current literature and a lack of consistency in recommended test procedure and interpretation . Sensitivity for peripheral nerve fiber damage ranged from 43% to 93% when using NCS as a reference standard . Both review papers did not recommend the sole use of monofilament testing to diagnose peripheral neuropathy . This is just one example of the shortcomings of current screening tests.
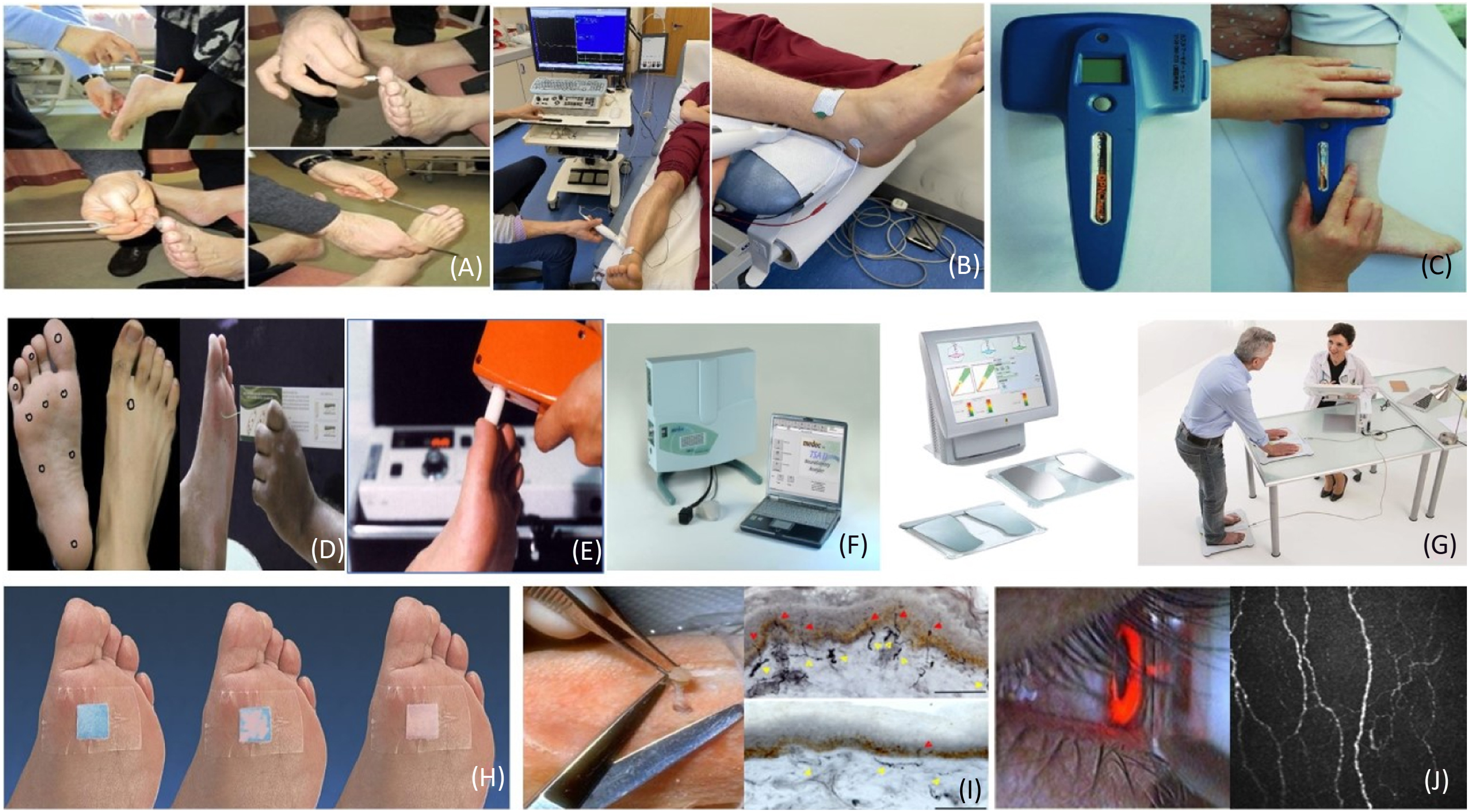
Diagnostic tests for diabetic peripheral neuropathy
While there is no single accurate definition of diabetic neuropathy, a simple definition for clinical practice is ‘the presence of symptoms and/or signs of peripheral nerve dysfunction in people with diabetes after excluding other causes’ . Based on this, the diagnostic tests are focused on assessing the symptoms and signs of nerve dysfunctions.
There are numerous testing methods available to assess the peripheral nervous system’s structure and function, with each test having its own advantages and disadvantages. Bedside tests used to aid diagnosis of DPN—including the 10 g monofilament ( Fig. 4.1D ), the Ipswich Touch Test, and vibration perception threshold testing with the Vibratip ( Fig. 4.1E ), a tuning fork, or automated devices such as Neurobiothesiometer, are not only reliant on patients’ subjective response but are also mainly used to identify the loss of protective foot sensation and risk of ulceration. As such, these tests tend to diagnose DPN when it is already well established. Late diagnosis hampers the potential benefits of intensified multifactorial intervention at an early stage of the disease, which could prevent the sequelae of DPN. Unfortunately, by the time DPN is detected with the crude tests currently used, it is often very well established and consequently impossible to reverse or halt the inexorable neuropathic process. Early diagnosis and timely intervention are thus essential in preventing the development of DPN.
Some of the most common tests and methods for diagnosis of DN have been summarized in Table 4.2 .
Nerve fibers assessed | Advantages | Limitations | ||
---|---|---|---|---|
Symptoms and signs | Questionnaires | Large (Aβ-fibers) and small (Aδ- and C-fibers) | Easy to administer. Used for monitoring symptoms | Lack of sensitivity, accuracy and reproducibility, subjective |
NDS | Large (Aβ-fibers) and small (Aδ- and C-fibers) | Does not require specialist equipment, assesses large- and small-fiber function | Not sensitive or reproducible, low correlation with small-fiber quantitative tests | |
10-g monofilament | Large (Aβ-fibers) | Simple, quick, and inexpensive . |
| |
Ipswich Touch Test | Large (Aβ-fibers) |
| Can only detect advanced neuropathy. | |
QST (Thermal and vibration thresholds) | Large (Aβ-fibers) and small (Aδ- and C-fibers) |
|
| |
Large-fiber tests | DPNCheck | Large, sural nerve (Aβ-fibers) | Quick, easy to perform, good sensitivity (92%–95%) compared to NCS | Relies on the accessibility of sural nerve . Validation studies had small patient numbers |
NCS | Large (Aβ-fibers) | A sensitive measure of large nerve function , reproducible | Doesn’t assess small fibers, uncomfortable , does not assess early neuropathic changes | |
Small-fiber tests | Skin biopsy (IENFD) | Small (C-fibers) | Gold standard for SNF, quantitative, good sensitivity, detects early nerve changes | Invasive, risk of infection, repeatability, requires trained personnel and special labs |
CCM | Small (Aδ- and C-fibers) | Noninvasive, good reproducibility , rapid and objective | Relatively expensive, requires specialist equipment and personnel, manual analysis is time-consuming | |
Autonomic tests | Neuropad | Small (C-fibers) |
| Varied interpretation of the results |
Sudoscan | Small (C-fibers) | Noninvasive, easy to perform |
| |
QSART | Small (C-fibers) | Sensitive for SFN (82%) , gold standard for measuring sudomotor function |
|
Symptoms
Various clinical scoring systems are available for DPN screening, including symptom scoring, sign scoring, or both ( Table 4.3 ). These systems may enhance diagnostic accuracy through a composite score of different combined tests and are useful tools for aiding diagnosis of DPN, along with quantitative measures. Each questionnaire has a scoring system that can diagnose, and in some, stratify disease severity. Table 4.3 presents a summary of the most commonly used questionnaires for assessing DPN.
Questionnaire | Assessed | Type of administration | Scoring | |
---|---|---|---|---|
Symptoms | NSP | Symptoms of neuropathy | Clinician administered |
|
DNS | Symptoms of diabetic peripheral neuropathy | Clinician administered | 4 for symptoms (Total 4) | |
NSS | Symptoms of neuropathy | Clinician administered |
| |
NPQ | Symptoms of neuropathic pain | Completed by the patient | Total of 12 | |
NPSI | Symptoms of neuropathic pain | Completed by patient | 10 descriptors, 2 duration (Total 12) | |
McGill Pain Questionnaire | Multidimensional symptoms of pain | Clinician administered |
| |
CNE | Signs of peripheral neuropathy | Clinician administered |
| |
Signs | NDS | Signs of peripheral neuropathy | Clinician administered |
|
DNE | Signs of peripheral neuropathy | Clinician administered |
| |
NIS-LL | Signs of neuropathy in the lower limbs | Clinician administered |
| |
MNDS | Signs of peripheral neuropathy | Clinician administered |
| |
UENS | Signs of peripheral neuropathy | Clinician administered | 11 for each side (Total 22) | |
Symptoms and signs | DN4 | Symptoms and signs of neuropathic pain | Clinician administered |
|
LANSS | Symptoms and signs of neuropathic pain | Clinician administered |
| |
TCNS | Signs and symptoms of peripheral neuropathy | Clinician administered |
| |
MNSI | Signs and symptoms of peripheral neuropathy | Symptoms by patient Foot examination by a clinician |
|
The Neurological Symptom Score (NSS) is a 17-question, interview-based assessment of sensory, motor, and autonomic function used to screen for DPN . Still, it is considered too extensive to be used efficiently in clinical practice. The diabetic neuropathy score (DNS) is an adaptation of the NSS that is a much quicker screening method, with only four questions and still offering moderate sensitivity (79%) and specificity (78%), but with slightly lower reliability for diagnosing DPN when using a diagnostic score of 1 or more.
Other symptom scoring systems focus only on pain and differentiating neuropathic from other causes. Clinicians commonly recognize pain descriptors that patients with neuropathic pain use. The McGill pain questionnaire was the first questionnaire designed to offer a multidimensional assessment of pain, which included assessing severity or intensity, emotional impact, and significance to the pain sufferer . This questionnaire is one of the most commonly used multidimensional pain scales globally. A short-form is available for screening, which has shown good agreement with the original version .
Signs
The Neuropathy Disability Score (NDS) is a commonly used clinical examination method that assesses neuropathy signs ( Fig. 4.1A ). Thirty-five items are used for both sides, evaluating cranial nerve damage, muscle strength, sensation loss and reflex delay/loss . However, some of the items have demonstrated only a weak relationship with DPN, and the full scoring system is too long to be used in clinical practice. Therefore a revised NDS has been created. This system is more commonly used and tests for four main neuropathy signs; ankle reflex, vibration, pinprick, and temperature sensation at both sides of the largest toes. A maximum score is 10, and usually, more than 6 is considered abnormal .
Composite scoring systems
The reliance on symptoms or signs alone may lead to low diagnostic accuracy for the presence of DPN, and a combination of both allows a more thorough assessment. Several scoring systems assess both signs and symptoms of DPN to produce a composite score. The Toronto clinical neuropathy score (TCNS) consists of three parts: symptom scores, reflex test scores and sensory test scores. The maximum score is 19, and the test is able to stratify patients into no DPN, mild DPN, moderate DPN, and severe DPN depending on the overall score . Testing has proven validity and reliability for diagnosing and staging DPN compared to electrophysiology measures .
The Michigan neuropathy screening instrument (MNSI) is another commonly used composite scoring system that includes a questionnaire and a foot examination . Neuropathy can be defined as seven or more positive responses to this symptoms section alone . The foot examination is more frequently used and encompasses foot appearance (including ulcers), ankle reflex, and the 128-Hz tuning fork test . One study found a range of sensitivity (35%–79%) and specificity (65%–94%) in comparison to NCS, depending on the cut-off value used for abnormality in MNSI. The higher specificity values indicate a potential high diagnostic impact for MNSI scoring; however, the lower sensitivity range indicates that milder DPN cases are likely not to get picked up.
Scoring of symptoms and signs is convenient and easy to perform as a method of screening for DPN. These tests are easily interpreted, making them a useful tool in supporting decisions on which patients should be referred for specialist assessment. Quantitative, objective measures should be considered when the patient has signs and symptoms other than those rated by the scoring test.
Large-fiber tests
Nerve conduction studies
The current “gold standard” for clinical diagnosis of DPN is through NCS by a trained neurophysiologist ( Fig. 4.1B ). In 2010, the Toronto Consensus, by an expert panel, recommended that one abnormal finding as part of NCS, combined with a symptom or sign of neuropathy, should be used to confirm DPN . NCS has also demonstrated an ability to predict future DPN .
For reliable NCS results, close attention must be paid to factors such as filter setting, limb temperature, and recording location, as outcomes can be vulnerable to variations. Trials have demonstrated that NCS consistently demonstrate excellent intraobserver agreement ; however, a poor interobserver agreement between expert clinical neurophysiologists is common when no standardized, specific technique is followed. One study assessed the results of four neurophysiologists from four different centers. Specific assessment methods were provided in a specially prepared syllabus, and a training session was provided beforehand. The outcome was a significant improvement in interobserver agreement with a standardized approach, and although not entirely eliminated, levels of disagreement were consequently considered clinically insignificant for medical practice .
Conversely, when considering the use of NCS in therapeutic clinical trials, even small interobserver variability may be significant enough to impact results through impacting the statistical power of a study and thus the trial’s outcomes. This may partially explain why previous clinical trials have used NCS as a primary outcome to detect treatment efficacy and have reported failed outcomes . Evidence supports the use of a single observer to repeat electrophysiological tests on each patient in these trials.
Furthermore, standard NCS testing is not easily applicable as a screening tool for DPN since it is time-consuming, requires a specialist operator and can be uncomfortable for the patient . Electrodiagnostic studies have also been identified as one of the largest drivers of health care costs related to neuropathy evaluation . Results are often found to be normal in patients with diabetes who have early or small-fiber predominant neuropathy.
DPNCheck
To overcome some of the shortcomings of standard NCS testing, a novel point-of-care nerve conduction device (POCD), DPNCheck (Neurometrix Inc., Waltham, MA) has been developed with the potential to serve as an acceptable proxy to standard NCS which are time-consuming, expensive, and often require patients to be seen in specialist clinics ( Fig. 4.1C ). This test for sural nerve conduction velocity and amplitude is much quicker (3 minutes) to perform than conventional electrodiagnostic testing. It has been validated in type 1 and 2 diabetes populations through comparison with the NDS and standard NCS . These studies have reported a high sensitivity of 92%–95% for detecting abnormalities ( Table 4.4 ). However, these studies’ cohorts have been small, with two of the three studies assessing very low numbers of patients with T1DM . Furthermore, the DPNCheck device is dependent on the presence of an accessible sural nerve which can be anatomically absent in up to 9% of healthy subjects .
Test | Fibers assessed | Validated against | Sensitivity | Specificity |
---|---|---|---|---|
DPNCheck | Large (Aβ-fibers) | NCS | 92%–95% | 82%–89% |
Neuropad | Small (C-fibers) | NCS, NDS, VPT | 70%–97.8% | 50%–67% |
Sudoscan | Small (C-fibers) | NCS, clinical examination, UENS, VPT, NSS | 70%–87.5% | 53%–92% |
CCM | Small (Aδ- and C-fibers) | NCS, clinical examination, CASS |
|
|
Small-fiber tests
Punch skin biopsy
The evidence strongly suggests that in DPN, damage to small fibers precedes damage to large fibers and punch skin biopsy is currently considered the gold-standard single test for diagnosing small-fiber neuropathy . A measure of intraepidermal nerve fiber density (IENFD) can be quantified from these biopsies, which is a method of documenting the density of terminal branches of peripheral nerves within the epidermis (no./mm 2 ). The European Federation of Neurological Societies has published guidelines for its use in diagnosing peripheral neuropathies ( Fig. 4.1I ).
Two immunostaining methods have become the most widely used in IENFD measurement: indirect immunofluorescence (IF) and bright-field immunohistochemistry (BFI). Although IF is considered a slightly more sensitive technique due to the higher signal/noise ratio , the two methods have excellent correlation , and both can comparably detect SFN . At present, age-related normative values exist only for BFI, published by a multinational group of eight centers .
For both IF and BFI techniques, IENFs are typically counted directly through an epifluorescence microscope’s oculars by focusing through the optical planes . For IF only, the more precise but time-consuming technique confocal microscopy (CM) can analyze optical sections of three-dimensional images using computer software . The two techniques have shown excellent correlation , and the latter is usually used when the more complex, second-level analysis is needed.
IENFD measurements have been shown to detect small-fiber neuropathy with depletion of IENFD detected in patients with normal NCS and no clinical signs or symptoms of neuropathy . A recent study reported low sensitivity of just 61% when using a cut-off of 4.5 fibers/mm IENFD to diagnose clinical DPN in T1DM patients . Earlier studies have published significantly higher values for sensitivity (80%) and specificity (95%) ; however, these studies were comparing healthy controls to DPN patients rather than the test’s ability to identify DPN in a diabetic cohort. Other studies have found a decrease in IENFD correlating with the progression of neuropathy and duration of diabetes , with reports that IENFD may also be lower in patients with painful DPN compared to painless DPN .
A 5-year follow-up study investigating the progression of DPN in T1DM and T2DM reported a significant reduction of IENFD in T2DM patients, with IENFD measurement being the single most abnormal parameter . Overall, the reduction in IENFD was not significant in T1DM subjects. However, the lower number of patients in the T1DM group may explain this finding, as it would make it more challenging to prove statistically significant changes .
The main issue with IENFD measurements as a biomarker for small-fiber neuropathy is that it is an invasive procedure. Obtaining a biopsy can cause side effects such as a mild infection due to improper wound management or, less commonly, excessive bleeding. Even though reported side effects are rare (1.9/1000) , the nature of this technique limits its practical use, particularly when a repeat biopsy is required in longitudinal studies or clinical intervention trials.
From a screening perspective, although IENFD measurement from a lower-limb skin biopsy is considered the gold standard for the diagnosis of small-fiber neuropathy, it is invasive and therefore not suitable for routine screening .
Quantitative sudomotor axon reflex test
The assessment of sudomotor nerve (sweat) function has also been used to assess small autonomic C-fibers, as anhidrosis can be characteristic of the presence of peripheral autonomic neuropathy.
The reference standard for measuring sudomotor function is the quantitative sudomotor axon reflex test (QSART). This test uses local sweat production, measured as a change of relative humidity over time, during and after skin activation. Special software is used to digitalize, plot, and analyses the temporal resolution, latency, magnitude, and duration of the sudomotor response . However, due to highly technical demands and relative discomfort of the examination, QSART remains mostly limited to research centers and is not considered a potential screening tool for DPN .
Neuropad
Neuropad is a patented 10-minute screening test for the early detection of diabetic foot syndrome and can be used as a triage test . It is a unique, noninvasive, painless, and simple diagnostic screening test employing a chemical reaction to minute quantities of sweat as a biomarker for much earlier signs of DPN.
The test has been created to assess the sweat function (small autonomic C-fibers) in the feet of patients with suspected neuropathy. An adhesive pad containing cobalt salts is stuck onto the foot’s plantar aspect and changes color from blue to pink within 10 minutes if the sudomotor function is normal . If there is a decreased function, the pad remains blue or turns patchy in color. There is a strong association between skin dryness, sudomotor dysfunction, and diabetic foot ulcer and the function of Neuropad. An abnormal Neuropad response is associated with sympathetic dysfunction and clinical neuropathy ( Fig. 4.1H ).
This test’s main advantage is that patients can self-administer at home, reducing clinical contact time and aiming to reinforce abnormal results visually. Instructions have been confirmed as clear for patients to follow, and the test is easy to use for most patients . However, due to older age, visual and kinetic problems, a fifth of patients still needed help when self-testing.
It has been reported as having good-to-excellent (70%–97.8%) sensitivity for DPN detection ( Table 4.4 ). When comparing Neuropad to a range of different small- and large-fiber diagnostic tests, the strong correlation between Neuropad and NDS , IENFD , CCM , Sudoscan , and measures of sweat gland dysfunction have been reported. It has also been identified as a useful tool for staging the severity of neuropathy in patients with type 2 diabetes, demonstrating excellent agreement with the Michigan classification system . Another significant advantage of Neuropad is its high NPV, making it ideal for serving as a screening test primarily to exclude DPN .
However, studies are not consistent in terms of the position of the Neuropad on the foot and the NDS cut-off value chosen to indicate clinical DPN presence. Furthermore, some studies graded the Neuropad color change as a percentage or score out of 1 , whereas others simply classified the results as normal or abnormal . Standardization of elapsed time before test result analysis is also necessary as extending the observation period to 15 minutes may provide greater diagnostic usefulness . This highlights a need for software development that can consistently grade each test’s color change over time to enable continuous and more accurate monitoring of sudomotor dysfunction.
To address these issues and increase both the sensitivity and specificity of Neuropad screening and create a continuous output, a smartphone software app and internet-based image-processing system has been developed. Neurometrics-Diab is a digital therapeutics (DTx) smartphone app that uses the Neuropad as a biomarker to produce a continuous record of a person’s neuropathy see if it is improving, is stable or is worsening with trend-lines helping to predict outcomes. Using a smartphone camera, patients can take a photo of their test result, which is then automatically sent to a web server where the photo is run through a proprietary image-processing algorithm resulting in a recorded percentage score. Over time a trend can be calculated. The DTx app is currently at the advanced prototype stage. Versions for other medical conditions are under development.
Sudoscan
Sudoscan (Impeto Medical) is another quick, simple, and noninvasive test that aims to assess sudomotor function using “reverse iontophoresis” to measure electrochemical skin conductance (ESC) of sweat in the hands and feet. Compared to age-corrected standard data, a reduced ESC result may indicate degeneration of small C-fibers that innervate the sweat glands and, therefore lead to reduced sweat gland function ( Fig. 4.1G ).
The ESC measurements from the feet are considered more sensitive for the detection of DPN than the hands , with less variation in results . This is likely due to a fluctuation in the hands’ contact on the electrodes. In contrast, the feet are aided by gravity to maintain constant pressure on the electrodes throughout the test. Lower ESC at the feet was also significantly associated with increasing symptoms in a large cohort of patients with T2DM .
Reference values in healthy subjects are available from a global collaborative analysis comparing different ethnic groups, age, and gender . This study noted a significantly lower hands and feet ESC for African–American, Indian, and Chinese populations than the Caucasian population, highlighting the need to match ethnicity groups in ESC studies. The same study also observed no significant difference between women and men at the hands or feet and a weak decline in ESC with increased age.
ESC measurements may also be associated with subjects’ weight , perhaps due to a weight-dependent change in sensitivity of the stainless-steel electrodes or sweat gland density when the subject is in the standing position. This could also be due to the correlation between higher weight and larger feet only . These hypotheses are yet to be assessed; however, these studies’ findings emphasize the importance of profile matching different subject groups for a weight that did not occur in some validation studies .
Validation studies have reported consistently good values for sensitivity (70%–87.5%) ( Table 4.4 ) when using foot ESC results to screen for DPN . However, there are inconsistencies in the ESC cut-off values used for identifying sudomotor dysfunction, ranging from 52 uS to 77 uS . This variation and inconsistencies in the neuropathy tests being used as a reference standard are the likely cause of the extensive range in reported specificity of between 53% and 92% . It highlights the need for standardization of the classification criteria used. Patient cohorts also differed in their severity of DPN, with participants in one study having significantly more advanced DPN than those in the study by Smith and colleagues . Therefore the test performed better in the former.
Overall, Sudoscan appears to be a promising DPN screening test that is noninvasive, easy to perform and eliminates the subjective component of clinician error, demonstrating a good correlation with IENFD . However, there is some doubt as the current evidence does not strongly support ESC to distinguish between patients with DPN and control individuals . Therefore longitudinal and more extensive cohort validation studies are needed, along with standardization of diagnostic criteria before Sudoscan can be used as a screening tool for small-fiber neuropathy.
Progress has been made in developing point-of-care devices (POCDs), which may diagnose DPN early before clinical signs are apparent. Neuropad, DPNCheck, and Sudoscan are newer screening tests that have demonstrated potential for early detection; however, validation studies, thus far, have reported a range of sensitivities and specificities depending on cohort and test used for comparison ( Table 4.3 ).
Quantitative sensory testing
Quantitative sensory testing (QST) has become a common method for evaluating small nerve fiber function using thermal threshold and thermal pain measurements and large-fiber function using vibration thresholds . The most common commercial system is the Medoc TSA-II NeuroSensory Analyser (Medoc Advanced Medical Systems, Israel) which is used to determine thermal thresholds ( Fig. 4.1F ). In recent years a cheaper, more portable device has been designed, NerveCheck (Phi Med Europe S.L., Barcelona, Spain), which has shown good reproducibility (ICC values=0.79, 0.71, and 0.86 for vibration, warm and cold sensation, respectively) and comparable diagnostic accuracy (86%, 72%, and 79% for vibration, warm and cold sensation testing, respectively) to established QST equipment for the diagnosis of DPN .
Cold thresholds can be used to evaluate myelinated Aδ-fiber function, whereas warm thresholds are used to assess the function of unmyelinated C-fibers. Published normative data sets are available for heat threshold detection , and recommendations for conducting QST in both clinical practice and research have previously been published by The International Association for the Study of Pain (NeuPSIG) .
QST has been found to have reasonable repeatability ; however, interoperator and interpatient variability depend on several factors. Training of both examiner and patient, the methodology of assessment, baseline skin temperature, stimulus characteristics, location and number of stimuli sites and duration of intervals between tests have all affected QST measurements . Using standardized methodology with extensive training has significantly reduced interobserver variability . However, this may be too time-consuming to be implemented.
When it comes to the effects of body fat on thermal detection thresholds, there are conflicting findings. Malmström et al. failed to detect differences between obese and other groups for cold and warm thresholds at the suprailiac site . In contrast, Price and colleagues found that obese participants had significantly higher cold and warm detection thresholds than normal BMI participants on the abdomen.
Two psychophysical algorithms can be used to determine thermal thresholds. These are the method of limits and the method of level (described in detail elsewhere) , with the method of limits used more commonly due to it being less time-consuming . Measurements determined using limits have been reported as significantly higher than those measured by level, irrespective of test location . However, the two methods correlate well with each other , and the 2013 consensus concluded that both were reliable . The major difference between these two methods is the effect of reaction time. For the method of limits, a patient has a longer reaction time due to age or height (causing a more extended sensory pathway), which may erroneously give a higher threshold.
Both warm and cold thresholds can be affected in patients with DPN, irrespective of how long the course of diabetes is, but the frequency of abnormal warm thresholds is significantly higher . A study found that cold detection thresholds significantly reduced in DM patients with no evidence of preclinical, subclinical, and clinical DPN, respectively . A longitudinal study also found a significant positive correlation between deterioration of cold detection thresholds and pain intensity in painful DN, with warm detection thresholds also correlating at nonsignificant value .
One major issue with the use of QST is that it cannot differentiate between peripheral and central temperature perception causes. It involves sensory receptors, spinal cord pathways and termination sites in the thalamus. This means that if there is poor concentration, a language barrier or cognitive defect, subjects’ results may affect their subjective nature .
Corneal nerves as a biomarker for diabetic peripheral neuropathy
Anatomically and developmentally, the eye can be considered an extension of the central nervous system (CNS). The cornea is the most densely innervated tissue in the body. It is richly supplied by a large number of sensory nerve fibers and a lesser number of autonomic fibers . The cornea possesses small unmyelinated C-fibers and myelinated Aδ-fibers for sensory innervation. These are derived from the trigeminal nerve’s ophthalmic division and enter the corneal stroma at its periphery, in a radial fashion parallel to the corneal surface. As the fibers run forward toward the cornea center, they lose their myelin sheath; a necessary step to maintain corneal transparency .
Corneal C-fibers form a delicate three-dimensional network known as the “subbasal nerve plexus” , which is located beneath the basal layer of the corneal epithelium. Mapping of the cornea has shown that this plexus forms a spiral or “whorl-like” pattern. The spiral center, often called the vortex, is located approximately 2–3 mm inferior and nasal to humans’ corneal apex. Due to this arrangement, subbasal nerves in the superior and human apical cornea are oriented vertically. In contrast, subbasal nerves in other corneal regions may be orientated horizontally or obliquely, consistent with their locations within the whorl-like plexus .
Corneal confocal microscopy (CCM) is a noninvasive, in vivo ophthalmic imaging technique that allows a detailed examination of the cornea, at high magnification, on a cellular level ( Fig. 4.1J ) . By capturing multiple two-dimensional images at different depths, CCM imaging can delineate the corneal layers , providing superior magnification compared to standard slit-lamp biomicroscopy. These properties allow CCM to acquire high-quality images of the corneal C-fibers in the subbasal nerve plexus. Considering the known relationship between damage to these fibers and DPN, the potential for their use as a surrogate biomarker for DPN has been identified.
When analyzing the subbasal nerve plexus, most studies report results from four morphological parameters: Corneal nerve fiber density (CNFD), which is the total number of main nerve fibers per mm 2 , corneal nerve fiber length (CNFL), which is the sum of the length of all nerve fibers and branches (mm/mm 2 ), tortuosity coefficient (TC) which is a unitless measurement that uses deviation from a straight line to measure the tortuosity of the main nerve fibers independent of their orientation, and corneal nerve branch density (CNBD) which is defined as the number of branches emanating from all main nerve fibers. There is, however, a discrepancy in how this can be quantified between studies with the established protocol for these parameters described elsewhere .
Of these four parameters, CNFL has been the most frequently used parameter for DPN, with one study reporting superior reliability than other parameters . Some studies have assessed the diagnostic performance of CCM for DPN and reported the results for CNFL only . Hertz et al. reported that CNFL produced the highest intra- and interobserver reproducibility (ICC of 0.72 and 0.73, respectively), with TC demonstrating the lowest (0.23 and 0.29, respectively).
Two other parameters that have been reported in research studies are nerve reflectivity and nerve fiber beading (no./100 µm) . Nerve fiber reflectivity is usually assessed using grades as first outlined by Oliveira-Soto and Efron , whereby classification can be split into four grades according to a comparison with reference images. The number of beadings is defined as the number of beadings in a length of 100 um of subbasal nerves within a frame . Both parameters have demonstrated changes in dry eye conditions, where patients with Sjogren’s syndrome have demonstrated significantly higher beading than dry eye patients of other primary causes . However, both measures require subjective judgment. Quantifying the beading is challenging, and it does require special software .
More recently, newer corneal parameters have been investigated. These include inferior whorl length (IWL) , defined as the length of the nerves at the inferior whorl of the superficial nerve plexus, nerve fiber width (CNFW) and nerve fiber area (CNFA) . These new measures have previously shown significant differences between the nonneuropathic and clinically neuropathic groups in DM with CNFW and CNFA, demonstrating 74% and 66% sensitivity–specificity equal error rate point, respectively when identifying nonneuropathic patients compared to control subjects . This indicates that these new measures may have the capacity to identify individuals with early neuropathy; however, research into these new parameters is currently limited.
Another type of cell found in the subbasal layer that has been of interest in DPN research are dendritic cells (DCs). These antigen-presenting cells of the cornea are of paramount importance. They play a critical role in activating other immune systems in the ocular surface, influencing both suppression and induction of inflammation .
Langerhans cells (LCs) are usually up to 15 μm in diameter and can be seen in various forms in the cornea . In their immature form, these cells have small dendritic processes or lack dendrites completely and are mainly located in the peripheral cornea’s epithelium . In pathological states, LCs mature, form interlocking dendritic processes, which may comprise a net-like structure, and migrate from the periphery into the central cornea .
Cross-sectional studies have shown an increase in the densities of LCs in the central cornea related to conditions such as dry eye with and without contact lens wear , bacterial keratitis , thyroid eye disease , and diabetes .
Corneal confocal microscopy for the detection of diabetic peripheral neuropathy
In the early 2000s, a novel study by Rosenberg and colleagues reported the correlation between increasing severity of DPN, corneal sensitivity and progressive loss of corneal subbasal nerve fibers . This was closely followed by a similar small study published in 2003 which found that CCM was able to detect abnormalities in the corneal nerves of 18 patients with diabetes deemed to have only mild neuropathy using conventional tests. Similarly, Midena and colleagues reported a significant decrease in corneal nerve fiber and branch number, along with decreased beading in patients with diabetes. It should be noted that these three studies used a light corneal confocal microscope, which is the first commercially available generation of the confocal imaging device with inferior image quality in comparison to the methods now commonly used.
Since then, the use of CCM for rapid, noninvasive clinical assessment of corneal nerves has grown substantially, especially in recent years. It has proven to be particularly useful as a diagnostic marker for detecting diabetic neuropathy and a range of other peripheral neuropathies . Some of them are reviewed in this paper.
Diagnostic performance for clinical diabetic peripheral neuropathy
Several cross-sectional studies have evaluated the ability of CCM to diagnose clinical levels of DPN in comparison to a range of other diagnostic tests ( Table 4.5 ). It must be noted that most of these studies assessed patients with T1DM only, meaning there is limited published data available for the diagnostic sensitivity and specificity values when assessing patients with T2DM.
