Keywords
Oncolytic virotherapy, brain tumors, gliomas, adenoviruses, autophagy, therapy, cancer
Introduction
Oncolytic virotherapy utilizes the ability of replicating viruses to selectively infect malignant cells, replicate within them, and subsequently lyse these cells to reinfect neighboring cells with the newly produced progeny. Various case reports in the previous century showed this paradigm to be a feasible alternative therapy for the treatment of cancer , and results from preclinical experiments for malignant gliomas using genetically modified tumor-specific oncolytic adenoviruses have been very promising .
The rationale for treating malignant gliomas with oncolytic adenoviruses is based on several aspects of the disease. First, no curative treatment options currently exist for patients diagnosed with malignant gliomas, and the potential for rapid recurrence after primary treatment necessitates the development of more efficient alternatives. Glioblastoma (grade IV glioma) has the most dismal prognosis, with a median survival of only 15 months after initial diagnosis despite the use of aggressive chemoradiotherapy regiments . Second, although oncolytic virotherapy for disseminated disease in several other cancer types is hampered by the physiologic boundaries of the circulatory and innate immune systems, metastasis is very uncommon in malignant gliomas; therefore, these tumors might be targeted using local delivery of oncolytic viruses. Last, molecular aberrations in several signaling pathways render malignant gliomas vulnerable to oncolytic adenoviruses and offer targets for the immune system.
Adenoviruses are double-stranded DNA viruses that can cause (usually mild) respiratory, digestive, and ocular infections in humans. Adenoviruses have long been used to deliver genes and are generally considered to be safe , easy to genetically manipulate , and researchers are capable of generating high-stock titers for clinical administration. However, adenoviral vectors were originally constructed to be replication deficient; thus, the therapeutic success depended on the efficacy of the first round of infection. This strategy later proved to be insufficiently potent for achieving a relevant anticancer efficacy , which led researchers to develop conditionally replication-competent adenoviruses as oncolytic therapy. Although the concept of inducing oncolysis by replicating vectors was not entirely new , the ability to modify the viral genome to increase safety, specificity, and modify infectivity led to a shift from gene therapy to oncolytic virotherapy.
Indeed, replication-competent adenoviruses proved to be more effective than replication-incompetent vectors for the treatment of solid tumors, thereby resulting in multiple clinical trials for several different tumor types . It is important to note that although 55 different human adenoviruses exist, all the current clinical trials ( n =87) of adenovirus-based therapy utilize (at least the backbone of) human adenovirus serotype 5 (Ad5) . This tendency is predominantly attributed to the commercial availability of convenient molecular tools for genetically modifying Ad5 and the extensive knowledge of this species genome. Although the basic genomic makeup of human adenovirus species is relatively well conserved, considerable interspecies variation exists at both ends of the genome in the E1 and E4 regions . The E1 region drives the replication of the virus, whereas the E4 region contains a variety of late genes that are, among other functions, involved in the capsid and binding motifs of the virus. Therefore, E4 variations may also account for the diversity between species in the tropism for specific cellular receptors for facilitating viral entry. Comparative studies have assessed the oncolytic efficiency of several human adenoviruses for treating animal models of melanoma, ovarian carcinoma, and lung carcinoma, and the oncolytic potential of Ad35 , Ad6, and Ad11 was shown to be similar to that of Ad5. Furthermore, chimeric vectors that utilize the Ad3 or Ad35 fiber for enhanced tumor tropism have been developed and used in glioma models and other malignancies. These chimeric viruses still depend on the Ad5 genes for nuclear trafficking, replication, and lysis; therefore, we focus on the Ad5 viral mechanisms.
Because viruses continuously adapt to manipulate and evade core cellular stress–response pathways to maintain their survival and reproduction, they can teach us important lessons about reverting the resistance of cancer cells to current cytostatic drugs. Furthermore, studying the ability of oncolytic viruses to subvert intracellular and systemic antiviral response can improve our understanding of the delicate interplay between tumor cells and their microenvironment.
The purpose of this chapter is to provide a basic understanding of the potential, caveats, and future directions of adenovirus-based virotherapy for malignant glioma.
Hurdles for Adenovirus-Based Oncolytic Virotherapy
The use of adenovirus for oncolytic virotherapy has been shown to have several pitfalls, many of which were identified during the testing of viral vectors designed for gene therapy. Prior to treatment, approximately 50% of the human population has been in contact with adenoviruses, with the generation of neutralizing antibodies that activate adaptive immune responses to the oncolytic adenoviruses soon after administration . The peripheral circulation forms a very effective barrier for adenoviruses because coagulation factors bind with high affinity to the capsid protein hexon . In addition, erythrocytes bind to adenoviruses through their coxsackievirus and adenovirus receptor (CAR) . Both phenomena result in an efficient sequestration of viral complexes into the liver and subsequent clearance. Although adenoviral clearance from the circulation is very effective, and therefore detrimental to an effective oncolysis, intratumoral delivery and its associated local immunogenicity may have ambivalent effects. In addition, the viral entry receptor CAR is highly expressed in a variety of off-target organs, whereas several tumors have been shown to have low or absent expression of CAR . In malignant gliomas, the expression levels of CAR and integrins are heterogeneous among and within individual patient samples and in conventional glioma cell lines , which were found to be predictive for the oncolytic efficacy. This aspect of CAR expression might limit both the specificity and the efficacy of oncolytic adenoviruses. Intriguingly, CAR has been shown to act as a tumor suppressor when introduced into the U118 glioma cell line in vitro and in flank tumors . Furthermore, recent publications have related CAR expression to α-catenin-induced migration and proliferation in colon cancer and have related the loss of CAR expression to chemotherapy-induced senescence .
Modifying Oncolytic Adenoviruses to Treat Malignant Gliomas
To overcome the previously described hurdles, researchers have evaluated alternative targeting strategies for redirecting oncolytic adenoviruses specifically to tumor cells while attenuating their ability to replicate and lyse healthy cells ( Table 10.1 ). Native adenoviral tropism is mediated by the fiber knob (attachment) and penton base (internalization) proteins in the capsid of the virus, which facilitate binding to CAR and integrin α V β 3 /β 5 , respectively. The following strategies for enhancing the tumor cell specificity of oncolytic adenoviruses have been explored: modification of the structural capsid to increase tumor tropism, deletion of viral early genes that are required for viral replication in off-target cells, and introduction of tumor tissue-specific promoters for viral replication . In the subsequent sections, we discuss the results of studies of oncolytic adenovirus for malignant glioma that have implemented these strategies.
Backbones | Agent | Tumor-Specific Promoter | Fiber Modification | Inserted Transgene | Additional Modification | References |
---|---|---|---|---|---|---|
Ad5 | Ad5-WT | VEGFR-1 | RGD | IL-24 | Kaliberova et al . | |
Midkine | Kohno et al . | |||||
pk7 | Zheng et al . | |||||
CAV1 knob | Zheng et al . | |||||
MAV-1 knob | Paul et al . | |||||
CAV2 knob | Paul et al . | |||||
Ad7 fiber | Paul et al . | |||||
CXCR4 | Ad3 knob | Ulasov et al . | ||||
Survivin | Ad3 knob | Ulasov et al . | ||||
GFAP | Ad35 | E4 under E2F promoter | Hoffman et al . | |||
COX-2 | Ad35 | E4 under Ki67 promoter | Hoffman et al . | |||
h-TERT | Ito et al . | |||||
HIF response element | dE3 | Post et al . | ||||
HIF response element | IL-4 | Cherry et al . | ||||
dE1A-E1B | Ad5-IR | Ad35 | TRAIL | Wohlfart et al . | ||
CB1 | Gomez-Manzano et al . | |||||
Delta-24 (dl922-947) | Delta-24 | Fueyo et al . | ||||
RGD | Suzuki et al . , Fueyo et al . | |||||
EGFRvIII ScFv | Y477A (abrogated CAR binding) | Piao et al . | ||||
TIMP3 | Lamfers et al . | |||||
hyCD | Conrad et al . | |||||
p53 | Geoerger et al . | |||||
Tyrosinase | Ad3 knob | E4 under tyrosinase promoter | Ulasov et al . | |||
E2F | RGD | Alonso et al . | ||||
dE1B55k (dl1520) | ONYX-015 | Geoerger et al . | ||||
Fk/20 | Shinoura et al . | |||||
Delo3 (dl520) | RGD | E1B19K | Holzmuller et al . |
Redirecting Adenoviruses Toward Glioma-Specific Receptors
Several strategies for caspid modification have been explored . Two of these were tested in the setting of malignant gliomas with varying success. The first strategy is based on the incorporation of ligands into the fiber protein, such as Arg-Gly-Asp (RGD) motif (binds to integrins), single-chain Fv fragment (scFV) antibodies (bind to tumor-specific receptors), or polylysine (binds to heparin receptors). The second strategy involves the creation of chimeric fiber-based vectors, which utilize the entry receptors of adenoviruses that naturally infect nonhuman species. A myriad of xeno-fibers have been reported ( Table 10.1 ).
Probably the most frequently and most extensively characterized approach for fiber modification in the context of malignant gliomas is the incorporation of an RGD motif into the HI loop of the fiber protein . This strategy was shown to enhance infectivity drastically in both conventional cell lines lacking CAR expression and primary patient-derived monolayers and organotypic spheroids . Retargeting oncolytic adenoviruses to the tumor-specific mutant epidermal growth factor receptor (EGFRvIII) also proved feasible in vitro and in vivo for targeting tumor cells expressing this receptor .
In an interesting comparative study of the infectivity of several fiber-modified vectors , the authors reported increased infectivity of vectors targeted toward the CD46 receptor, which is the preferred entry receptor for many type B adenoviral species (e.g., Ad35 but not Ad3). This receptor facilitates the cleavage of complement factors C3b and C4b to prevent the activation of the complement system against the host cell . Although it was first discovered in monocytes, expression of the CD46 receptor is more or less ubiquitous; the CD46 receptor is shared among numerous pathogens as an entry receptor, and its role in oncolytic measles virus cell entry has been studied extensively . CD46 expression in malignant gliomas is still somewhat controversial , although most studies have confirmed that chimeric fibers that supposedly utilize this receptor displayed and enhanced infectivity in a subset of malignant gliomas cultures. Particularly, the infectivity in early passage primary glioblastoma cell cultures was markedly improved for the chimeric Ad5/35 vector compared to the wild-type Ad5 .
Unlike Ad5/35, Ad5/3 chimeric vectors have been shown to utilize the CD80/86 receptor and similarly enhance infectivity compared to wild-type Ad5. Furthermore, these vectors were found to be less cytotoxic in normal human astrocyte cultures . Xeno-fiber chimeric vectors based on several different adenoviral species were tested in both conventional glioma cell lines and patient-derived glioblastoma cultures . The authors of that study could not confirm the success of Ad5/3 vector infectivity in primary glioblastoma samples; however, they did find striking increases in the infectivity by canine- and porcine-derived xenotype fiber vectors. Taken together, all these studies confirm the feasibility of increasing the therapeutic efficacy of Ad5 backbone-based vectors by modifying the method of cell entry.
One important caveat has been reported in one study, in which the authors were unable to reproduce the in vitro beneficial effects of a panel of capsid-modified viruses when tested in xenograft glioblastoma models with either low- or high-CAR expression . By measuring transduction of a luciferase imaging system as derivative for infectivity, the authors found that the modified adenoviral vectors were not superior to the unmodified adenoviral vector in D54-derived xenografts (high CAR) or U87-derived xenografts (low CAR). This study underscores the limitations of in vitro models for predicting infectivity of adenoviruses, and it suggests that the role of the extracellular matrix and hypoxia should be considered as relevant factors that contribute to therapeutic success (or the lack thereof) in xenografts. In addition, none of these studies tested these vectors in glioma stem cell (GSC)-based models. In approximately the past decade, GSCs have been established as a superior model for studying glioblastoma drug response—compared to conventional cell lines such as U251, U87, and U373—in regard to the maintenance of glioblastoma gene expression signatures and recapitulation of glioblastoma hallmarks in murine xenograft models . One study did examine CAR expression in a panel of four GSC cultures and found that 60–95% of cells in these cultures were positive, thereby leading to effective oncolysis with Delta-24-RGD and a prolongation of survival from 38.5 to 66.3 days in a GSC-derived xenograft model . Nonetheless, the infectivity of GSCs remains to be interrogated for CD46-, CD80-, and CD86-targeted vectors.
Targeting Cancer Genetic Pathways
The second well-established strategy for creating tumor specificity is the implementation of genetic modifications that attenuate the replication of oncolytic adenoviruses in nonmalignant cells. Adenoviruses hijack the function of several tumor-suppressive pathways, which are inactivated in cells in the G 0 cell cycle phase, to facilitate the efficient transcription of viral genes. This viral manipulation of the cellular machinery is facilitated by the adenoviral E1A gene, and it is performed in concert with the prevention by the adenoviral E1B genes of the subsequent cellular activation of apoptosis signaling pathways . In malignant gliomas, both cell cycling and apoptosis pathways are frequently dysfunctional and are thereby putative candidates for adenoviral replication. The suggested paradigm here would be the modification of the viral genome targeting a neoplastic pathway.
Indeed, the first oncolytic adenovirus tested in the context of malignant gliomas was the E1B-55K-deleted (dl1520) ONYX-15 virus. E1B-55K binds to p53 in the early stage of infection, thereby effectively blocking apoptotic signaling induced by the transduction of the viral E1A gene . Approximately 35% of glioblastomas harbor somatic mutations in the p53 gene, whereas 87% have altered signaling within the p53 cascade . Because binding to p53 is not the only function of E1B-55K, other (at least equally) important factors are likely contributing to the tumor selectivity of ONYX-15 . ONYX-15 was the first oncolytic adenovirus to be tested in a clinical trial, which demonstrated a favorable toxicity profile for both intratumoral and intravascular administration. Imaging studies and lymphocytic infiltration suggested ONYX-15 had biological activity; however, the clinical benefit to patients was not significant . The lack of clinical evidence for ONYX-15 as an effective agent was recently countered by the approval of the Chinese Food and Drug Administration for the treatment of refractory head and neck cancer in combination with cisplatin after a successful phase III trial. These results warrant the exploration of multimodal treatment strategies, which we discuss later in this chapter.
The cell cycle-targeted variant of this strategy is successfully capitalized by a 24-bp deletion in the CR2 of E1A coined Delta-24 , and a similar vector was reported as dl922-947 . This deletion results in the inability of E1A to bind to the retinoblastoma protein, a protein essential for cell growth arrest in the G 1 phase, by blocking the transcription factor E2F1. By doing so, E1A is causing the release of the transcriptional activity of E2F; as a consequence of the release of E2F, there will be activation of cellular pathways that are critical for viral replication . The retinoblastoma-related network is altered in approximately 78% of glioblastomas, and homozygous deletions of retinoblastoma gene are found in 11% of them, according to The Cancer Genome Atlas (TCGA) data set . The Delta-24 mutation has been implemented in several vectors investigated in preclinical studies of malignant gliomas . Mechanistically, the small 24-bp alteration does not affect the maximum evolutionarily conserved adenoviral machinery, therefore maintaining the oncolytic potency of the agent while leaving room for the insertion of potentiating transgenes. This biological agent is being clinically evaluated for the first time in a phase I trial investigating the biosafety of intratumoral injection (opened December 2008; ClinicalTrials.gov ID No NCT00805376). A second independent trial was initiated to investigate convection-enhanced delivery via 48 hr of continuous infusion (EudraCT No. 2007-001104-21). At the time of writing of this chapter, both trials were still accruing patients.
Another vector that has been studied for treating malignant gliomas is Ad5-YB1 (also termed dl520). This oncolytic adenovirus depends on the nuclear accumulation of the transcription factor YB-1 for efficient replication. Adenoviral DNA replication is facilitated by the interplay between E1A-E2F and the E2 viral promoters. E2 expression is regulated by E1 and E2 late promoters. The nuclear accumulation of YB-1 facilitated by E1B-55k (which can be transcribed independent of E1A after high viral dosing) has been demonstrated to induce the expression of the E2 late promoters, thereby facilitating viral DNA replication . Ad5-YB1 can therefore be used as an oncolytic adenovirus to facilitate E1A-independent viral replication and, consequently, oncolysis . Elevated nuclear YB-1 expression was demonstrated in glioblastomas . Furthermore, YB-1 expression was particularly high in glioblastoma vasculature , and Ad5-YB-1 seemed to repress vascular endothelial growth factor levels in glioblastoma cell lines in vitro . Ad5-YB1 can therefore be used as an oncolytic adenovirus to facilitate E1A-independent viral replication and, consequently, oncolysis .
Tumor-Specific Promoter-Driven Oncolytic Adenoviruses
Similar to the previously described strategy, the tumor-specific promoter (TSP)-driven oncolytic adenoviruses mainly derive their specificity from withholding the ability to replicate in nonmalignant cells. All the oncolytic adenoviruses that have been evaluated in studies of glioblastoma models have linked E1A function to a promoter that is active in tissue and/or tissue vasculature ( Table 10.1 ). For oncolytic adenoviruses, the TSP should be homogenously expressed and at a higher rate in tumor tissue than in normal brain tissue and in other organs such as the liver in order to minimize toxicity and facilitate the efficient replication of the viral genome. Unfortunately, the number of promoters that fulfill these requirements is limited. Therefore, combinations of safety strategies are implemented, such as using the backbone Delta-24 vector with the E2F response element as applied in the oncolytic adenoviruses named ICOVIR .
Hypoxia/hypoxia-inducible factor (HIF)-dependent replicative adenoviruses (HYPR-Ads) are controlled by hypoxia-inducible response elements, leading to transcription of E1A in hypoxic cells , resulting in specific lysis of glioblastoma, medulloblastoma, and normal cells under hypoxic conditions in vitro . Although the ability of oncolytic adenoviruses to replicate in hypoxic tumors seems to be problematic, both wild-type adenovirus and the HYPR-Ads were able to replicate under hypoxic conditions. HYPR-Ads were also shown to replicate in hypoxic tumor areas in a xenograft flank model of the LN229 glioblastoma cell line . Novel improved oncolytic adenoviruses based on the same principle with augmented replicative ability (HIF-Ad) have been combined with interleukin-4 (IL-4) to boost adaptive immune response .
Another specific feature of tumor cells is telomerase expression; therefore, the human telomerase reverse transcriptase (hTERT) promoter is specifically active in malignant cells. The use of the hTERT promoter to direct E1A transcription was first reported in glioblastomas by Ito et al . . Other promoters that have been utilized in glioblastomas are the E2F , midkine , survivin , CXCR4 , tyrosinase , and vascular endothelial growth factor promoters. One of the few comparative studies of TSP-driven oncolytic adenoviruses showed that survivin was superior to CXCR4 and midkine with regard to both tumor-specific expression and oncolytic efficacy in vitro .
The true specificity of promoter-driven E1A transcription is debatable because only very few copies of E1A can lead to viral replication and subsequent E1A accumulation . To circumvent this problem, researchers have developed double-promoter systems that lock the transcription of E4 under a second specific promoter. This strategy was applied to glioblastoma in a study by Hoffmann et al . , in which two vector systems were shown to work particularly well. These authors used a combined system that was designed using the Delta-24 background with E1A transcription regulated by the GFAP/COX-2 (cyclooxygenase-2) tissue-specific promoter, followed by a proliferation-specific E4 transcription (using E2F/Ki67 promoter ). This double heterologous system proved to be selective and effective in a panel of cell lines. Moreover, in combination with a chimeric fiber (Ad5/35), the use of this system rendered significantly longer survival times compared to the homologous Ad5-based counterparts in a subcutaneous glioblastoma xenograft model .
In summary, the safety and specificity of oncolytic adenoviruses in vivo have been favorable thus far. This does not imply that incorporating extra safety mechanisms, without hampering therapeutic effect, is futile. Combining the aforementioned three strategies should accommodate the development of clinically secure vectors. Of note, in other solid tumors, the use of tissue-specific microRNAs has proven to be a useful strategy for targeting oncolytic adenoviruses to tumor cells efficiently . This strategy has not yet been tested in glioblastomas, although miRNAs have been suggested to play an important role in the development of the disease, which would facilitate the development of glioblastoma subtype-specific oncolytic adenoviruses .
Systemic Delivery of Oncolytic Adenoviruses by Cellular Vehicles
The first trials with replication-incompetent adenoviruses revealed only limited spread of these vectors from the site of administration . Therefore, the paradigm of reinfection after efficient lysis by replication-competent adenoviruses was thought to improve the dissemination of the oncolytic adenoviruses. This notion seemed to hold true in animal models, but it remains to be confirmed in clinical trials. Because oncolytic adenoviruses are administered intratumorally, the blood–brain barrier is less relevant than when agents are intravenously administered. However, it remains to be discerned how efficiently oncolytic adenoviruses can disperse through the tumor parenchyma and infect invasive tumor cells in patients. Factors such as (altered) extracellular matrix, hypoxia, necrosis, and perturbed intratumoral perfusion have all been described to negatively influence viral spread . Furthermore, intratumoral administration limits the dosing frequency of oncolytic adenoviruses to a singular or once-repeated event, which should ideally take place after tumor debulking.
Several strategies have been explored to circumvent this potentially limiting factor. The carrier cell (or Trojan horse) strategy has been applied in the context of oncolytic adenoviruses by infecting neural , mesenchymal , and adipose stem cells for systemic or intratumoral delivery. For other oncolytic adenoviruses, leukocytes have been successfully employed as well . Once infected, carrier cells have (1) the capability to shield viral particles from the peripheral immune barriers, (2) tropism toward the tumor microenvironment, and (3) the ability to produce progeny to facilitate the amplification of viral release into the tumor microenvironment. This strategy potentially switches the administration of virus from locally to systemically, allowing for more frequent and timed dosing.
Other enhanced features of vehicles that have been explored include the ability to contribute to an antitumor immune response and the secretion of therapeutic agents. Stem cells can be manipulated to deliver several therapeutic agents (e.g., miRNAs, cytokines, and prodrugs); however, they are thought to have some potential disadvantages, such as malignant degeneration after grafting and negative contributions to the immune-suppressive or angiogenic tumor environment . Loading stem cells with oncolytic adenoviruses resolves these problems because the vehicle cell will succumb to the infection. The tropism of carrier cells in general, before and after being loaded with oncolytic adenoviruses, demands special attention because not every in vivo model seems to be equally well attractive for homing purposes. The feasibility of this strategy was first demonstrated by the observation that neural stem cells had the capability of migrating from the contralateral hemisphere to the ipsilateral hemisphere harboring a xenograft tumor .
Several studies have demonstrated that once these vehicles get into the tumor, the delivery of oncolytic adenovirus is accomplished, resulting in therapeutic efficacy . Doucette et al . demonstrated the homing capacities of murine mesenchymal stem cells (MSCs) delivered via intracarotid injection toward a PDGF-B-induced RCAS/NTVa model . These MSCs were not infected with virus, and therefore the results have no predictive value with regard to addressing the ability of these cells to shield the virus from the circulation. Other researchers have reported that the delivery of MSCs via intravenous injection may yield lower intratumoral delivery than delivery via intracarotid injection . In a different comparative study, the efficiency of neural stem cells to deliver oncolytic adenoviruses was found to be superior to that of MSCs in U87-derived xenografts . More mechanistic studies are needed to elucidate the molecular factors that contribute to the homing of stem cells toward glioblastoma models.
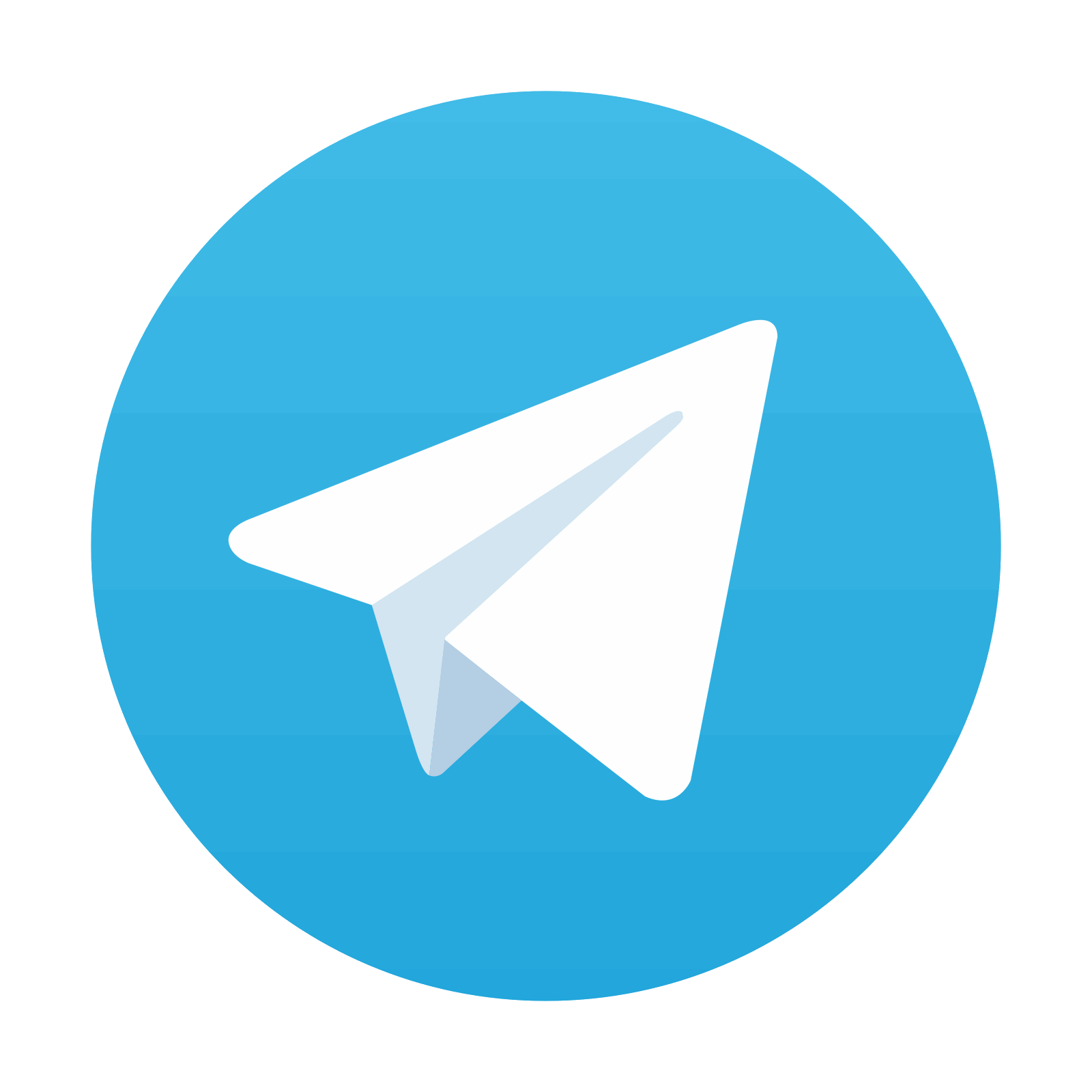
Stay updated, free articles. Join our Telegram channel

Full access? Get Clinical Tree
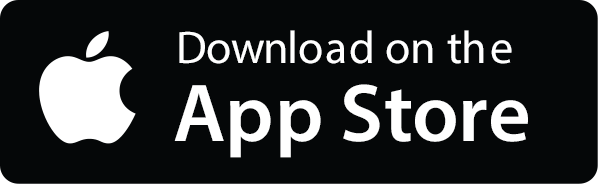
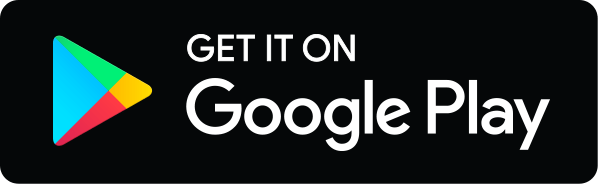