INTRODUCTION
Acute myeloid leukemia (AML) consists of a heterogeneous group of hematologic neoplasms characterized by clonal proliferation of myeloid blasts in the peripheral blood, bone marrow, and extramedullary tissues. Despite advances in our understanding of the molecular biology of AML, its treatment remains challenging and outcomes vary greatly depending on the cytogenetic and molecular features as well as age and comorbidities.
Acute myeloid leukemia is thought to be the culmination of genetic mutations and chromosomal aberrations within myeloid precursors resulting in disrupted differentiation, excessive proliferation, and suppressed apoptosis of neoplastic cells referred to as blasts.
Over the last several decades, improvements in chemotherapeutic regimens and supportive care have resulted in significant but modest progress in treating AML. Better understanding of the biology of AML has resulted in the identification of new therapeutic targets. Despite this, currently, the majority of patients with AML die from the complications of their disease. With better definition of molecular abnormalities and elucidation of the pathogenic events in various AML subtypes and with the development of novel targeted agents, a better outcome for patients with AML may be achievable in the future.
EPIDEMIOLOGY, ETIOLOGY, AND RISK FACTORS
Approximately 13,000 individuals are diagnosed annually in the United States with leukemia. The incidence of AML is 4.3 per 100,000 (1). The median age at presentation is about 65 years. The incidence of AML, as well as myelodysplastic syndrome (MDS), appears to be rising, particularly in individuals over 60 years of age. The incidence of AML is slightly higher in males and in populations of European descent. Acute promyelocytic leukemia (APL), a distinct subtype of AML, has been reported to be more common among populations of Hispanic background (2).
An increased incidence of AML is seen in patients with disorders associated with increased chromatin fragility such as Bloom syndrome, Fanconi anemia, Kostmann syndrome, and Wiskott-Aldrich syndrome or ataxia-telangiectasia. Other syndromes, such as Down (trisomy of chromosome 21), Klinefelter (XXY and variants), and Patau (trisomy of chromosome 13) syndromes, have also been associated with a higher incidence of AML (3).
Therapeutic radiation increases AML risk, particularly if given concomitantly with alkylating agents. Two categories of therapy-related AML have been described. Patients exposed to alkylating agents (eg, cyclophosphamide, melphalan, nitrogen mustard) can develop AML after a latency period of 4 to 8 years, which is often associated with abnormalities of chromosomes 5 and/or 7. Exposure to agents that inhibit the DNA repair enzyme topoisomerase II (eg, etoposide) is also associated with secondary AML with a shorter latency period, usually 1 to 3 years (4). Benzene, smoking, dyes, herbicides, and pesticides have been implicated as potential risk factors for development of AML (5).
Acute myeloid leukemia may also be secondary to transformation of an antecedent myeloid disorder, such as MDS, myeloproliferative neoplasm (MPN), or MDS/MPN, or other bone marrow disorders, such as aplastic anemia.
CLINICAL PRESENTATION
Fatigue, bruising or bleeding, fever, and infection, reflecting a state of bone marrow failure, are common in AML. Only 10% of patients present with white blood cell (WBC) count greater than 100 × 109/L (1). These patients are at higher risk of tumor lysis syndrome, central nervous system involvement, and leukostasis. Leukostasis may manifest as dyspnea, chest pain, headaches, altered mental status, cranial nerve palsies, or priapism. Leukostasis and tumor lysis syndrome are oncologic emergencies and require prompt recognition and management.
Physical findings other than bleeding and infection may include organomegaly, lymphadenopathy, sternal tenderness, retinal hemorrhages, and infiltration of gingivae, skin, soft tissues, or meninges (more common with monocytic variants, M4 or M5). Disseminated intravascular coagulopathy (DIC) with bleeding diathesis is a common presentation in APL.
DIAGNOSIS AND CLASSIFICATION
The diagnosis of AML is typically based on the presence of 20% myeloid blasts in the bone marrow or peripheral blood in accordance with World Health Organization (WHO) classification criteria (Table 2-1) (6). Acute myeloid leukemia subtypes in the WHO classification are defined on the basis of morphology, immunophenotype, and molecular/genetic features. In some patients, AML presents as a mass in extramedullary tissues (myeloid sarcoma). Patients who have the cytogenetic abnormalities t(8;21)(q22;q22), inv(16)(p13q22) or t(16;16)(p13;q22), and t(15;17)(q22;q12) are diagnosed with AML regardless of the blast percentage.
Myeloproliferative neoplasms (MPN) Chronic myelogenous leukemia, BCR-ABL1–positive Chronic neutrophilic leukemia Polycythemia vera Primary myelofibrosis Essential thrombocythemia Chronic eosinophilic leukemia, not otherwise specified Mastocytosis Myeloproliferative neoplasms, unclassifiable Myeloid and lymphoid neoplasms associated with eosinophilia and abnormalities of PDGFRA, PDGFRB, or FGFR1 Myeloid and lymphoid neoplasms associated with PDGFRA rearrangement Myeloid neoplasms associated with PDGFRB rearrangement Myeloid and lymphoid neoplasms associated with FGFR1 abnormalities Myelodysplastic/myeloproliferative neoplasms (MDS/MPN) Chronic myelomonocytic leukemia Atypical chronic myeloid leukemia, BCR-ABL1–negative Juvenile myelomonocytic leukemia Myelodysplastic/myeloproliferative neoplasm, unclassifiable Provisional entity: refractory anemia with ring sideroblasts and thrombocytosis Myelodysplastic syndrome (MDS) Refractory cytopenia with unilineage dysplasia Refractory anemia Refractory neutropenia Refractory thrombocytopenia Refractory anemia with ring sideroblasts Refractory cytopenia with multilineage dysplasia Refractory anemia with excess blasts Myelodysplastic syndrome with isolated del(5q) Myelodysplastic syndrome, unclassifiable Childhood myelodysplastic syndrome Provisional entity: refractory cytopenia of childhood Acute myeloid leukemia and related neoplasms Acute myeloid leukemia with recurrent genetic abnormalities AML with t(8;21)(q22;q22); RUNX1-RUNX1T1 AML with inv(16)(p13.1q22) or t(16;16)(p13.1;q22); CBFB-MYH11 APL with t(15;17)(q22;q12); PML-RARA AML with t(9;11)(p22;q23); MLLT3-MLL AML with t(6;9)(p23;q34); DEK-NUP214 AML with inv(3)(q21q26.2) or t(3;3)(q21;q26.2); RPN1-EVI1 AML (megakaryoblastic) with t(1;22)(p13;q13); RBM15-MKL1 Provisional entity: AML with mutated NPM1 Provisional entity: AML with mutated CEBPA Acute myeloid leukemia with myelodysplasia-related changes Therapy-related myeloid neoplasms Acute myeloid leukemia, not otherwise specified AML with minimal differentiation AML without maturation AML with maturation Acute myelomonocytic leukemia Acute monoblastic/monocytic leukemia Acute erythroid leukemia Pure erythroid leukemia Erythroleukemia, erythroid/myeloid Acute megakaryoblastic leukemia Acute basophilic leukemia Acute panmyelosis with myelofibrosis Myeloid sarcoma Myeloid proliferations related to Down syndrome Transient abnormal myelopoiesis Myeloid leukemia associated with Down syndrome Blastic plasmacytoid dendritic cell neoplasm Acute leukemias of ambiguous lineage Acute undifferentiated leukemia Mixed phenotype acute leukemia with t(9;22)(q34;q11.2); BCR-ABL1 Mixed phenotype acute leukemia with t(v;11q23); MLL rearranged Mixed phenotype acute leukemia, B-myeloid, NOS Mixed phenotype acute leukemia, T-myeloid, NOS Provisional entity: natural killer (NK) cell lymphoblastic leukemia/lymphoma B lymphoblastic leukemia/lymphoma B lymphoblastic leukemia/lymphoma, NOS B lymphoblastic leukemia/lymphoma with recurrent genetic abnormalities B lymphoblastic leukemia/lymphoma with t(9;22) (q34;q11.2);BCR-ABL 1 B lymphoblastic leukemia/lymphoma with t(v;11q23); MLL rearranged B lymphoblastic leukemia/lymphoma with t(12;21) (p13;q22) TEL-AML1 (ETV6-RUNX1) B lymphoblastic leukemia/lymphoma with hyperdiploidy B lymphoblastic leukemia/lymphoma with hypodiploidy B lymphoblastic leukemia/lymphoma with t(5;14) (q31;q32) IL3-IGH B lymphoblastic leukemia/lymphoma with t(1;19) (q23;p13.3);TCF3-PBX1 T lymphoblastic leukemia/lymphoma |
Bone marrow sampling is essential for the initial workup of a patient with suspected acute leukemia. Sampling should include a core biopsy as well as aspiration material. The core biopsy should be used to perform touch preparations, which are invaluable in case of a dry tap. The aspiration material is used to prepare a clot and aspirate smears, in addition to having portions submitted for flow cytometry, cytogenetics, and molecular diagnostics.
Confirmation of myeloid lineage is commonly accomplished using flow cytometry. In most AML cases, blasts express one or more markers of immature hematopoietic precursors such as CD34 and HLA-DR, in addition to dim CD45 (common leukocyte antigen). Myeloid lineage is predicated on the expression of antigens associated with granulocytic, monocytic, erythroid, and/or megakaryocytic differentiation by blasts (Table 2-2). Expression of myeloperoxidase (MPO) is considered specific for myeloid differentiation, although AML blasts may lack MPO expression. Although aberrant expression of lymphoid antigens is commonly seen in some cases with a bona fide AML phenotype, such expression is generally limited to one or a few lymphoid antigens. Acute leukemia with ambiguous lineage is beyond the scope of this discussion; briefly, it refers to acute leukemia with overlapping expression of myeloid and lymphoid antigens or lack of both.
Molecular diagnostics play a critical role in the laboratory workup of AML. Whereas the scope of known molecular alterations in AML was limited until a few years ago, the advent of next-generation sequencing (NGS) has dramatically increased our understanding of the molecular landscape of AML. As a result, the limited number of polymerase chain reaction (PCR)–based assays that used to be performed during AML workup have been gradually replaced at most large facilities by NGS-based panels that simultaneously assess the mutation status of tens to hundreds of genes. The current NGS mutation panel for new and relapsed myeloid malignancies used at our institution assesses the coding sequences of the 28 genes listed in Table 2-3.
ABL1 | EGFR | GATA2 | IKZF2 | MDM2 | NOTCH1 | RUNX1 |
ASXL1 | EZH2 | HRAS | JAK2 | MLL | NPM1 | TET2 |
BRAF | FLT3 | IDH1 | KIT | MPL | NRAS | TP53 |
DNMT3A | GATA1 | IDH2 | KRAS | MYD88 | PTPN11 | WT11 |
RISK STRATIFICATION OF ACUTE MYELOID LEUKEMIA
Once a diagnosis of AML has been made, the next step is to risk stratify the patient. Several variables are predictive of outcome, including patient-related variables, such age and performance status, as well as disease-related predictors, such as cytogenetic and molecular characteristics.
The karyotype remains one of the best predictors of outcome in patients with AML. The European LeukemiaNet (ELN) guidelines have proposed a risk stratification system based on cytogenetics and molecular analysis (7). As listed in Table 2-4, the patients are classified as having favorable-, intermediate-, and unfavorable-risk disease. The drawback of this classification system is that it takes into account few mutations for prognostication. More recent data suggest that the favorable prognosis attributed to mutant CEBPA is true in cases of bilallelic CEBPA mutation rather than the monoallelic mutation (8). Of note, in patients with FLT3-negative/NPM1-positive AML, DNMT3A assessment is of importance. Loghavi et al showed that in patients with karyotypically normal AML with NPM1 mutations, presence of concurrent DNMT3A mutation has an adverse effect on outcomes, with the effect being more detrimental than either FLT3-ITD or FLT3-TKD mutations (9). This analysis showed that of patients with de novo karyotypically normal AML, particularly those <60 years old, AMLDNMT3A/FLT3/NPM1 patients seem to have the worst clinical outcomes, followed by those with AMLFLT3/DNMT3A and then those with AMLNPM1/DNMT3A (9). Currently, normal karyotype AML patients with NPM1-positive/FLT3-negative disease are considered favorable risk according to the ELN guidelines.
Genetic group | Subsets |
---|---|
Favorable | t(8;21)(q22;q22); RUNX1-RUNX1T1 |
inv(16)(p13.1q22) or t(16;16)(p13.1;q22); CBFB-MYH11 | |
Mutated NPM1 without FLT3-ITD (normal karyotype) | |
Mutated CEBPA (normal karyotype) | |
Intermediate-Ia | Mutated NPM1 and FLT3-ITD (normal karyotype) |
Wild-type NPM1 and FLT3-ITD (normal karyotype) | |
Wild-type NPM1 without FLT3-ITD (normal karyotype) | |
Intermediate-II | t(9;11)(p22;q23); MLLT3-MLL |
Cytogenetic abnormalities not classified as favorable or adverseb | |
Adverse | inv(3)(q21q26.2) or t(3;3)(q21;q26.2); RPN1-EVI1 |
t(6;9)(p23;q34); DEK-NUP214 | |
t(v;11)(v;q23); MLL rearranged | |
–5 or del(5q); –7; abnl(17p); complex karyotypec |
The presence of TP53 mutations is associated with a poor outcome. A recent report by German/Austrian investigators indicated that the outcome of patients with TP53 mutations is worse than that of patients with the monosomal karyotype known to be an adverse prognostic indicator (10). In this study, the analysis showed that the TP53 mutation was commonly associated with older patients and a monosomal karyotype and correlated with a low complete remission (CR) rate and shorter relapse-free survival (RFS), event-free survival (EFS), and overall survival (OS). On multivariate analysis, TP53 mutations were associated with the worst prognosis (10).
Several novel molecular aberrations, including mutations of the IDH, ASXL1, DNMT3A, TET2, MLL, and PHF6 genes, have been described, particularly in patients with a normal karyotype (11). However, their utility in clinical practice remains limited, and they have limited value in determining best treatment strategies for patients.
TREATMENT OF ACUTE MYELOID LEUKEMIA
In the 1960s, Freireich et al demonstrated the significance of achieving a CR to improve survival (12). Since then, the objective of therapy has been to produce and maintain CR, the only currently accepted approach to AML cure. Criteria for CR have been defined by the International Working Group and are followed in the clinic and in clinical trials for response assessment (13). After 3 years in CR, the probability of AML recurrence sharply declines to less than 10% (14), and patients in continuous CR for 3 or more years can be considered “potentially cured.” However, clearly the definition of CR is an arbitrary one, and with improved technology, the precision for detection of residual disease after the initial therapy has increased. Whether this improved detection and application of novel strategies to eradicate the residual leukemia will translate to improved cure rates will be the subject of future trials.
Once AML is diagnosed, the need for emergency therapy must be assessed. Emergency treatment is required (1) in cases of APL, (2) if the circulating blast count is >50 to 100 × 109/L, and (3) in the presence of DIC or organ dysfunction (especially pulmonary) attributed to leukemic infiltration (mostly seen in patients with >10 × 109/L circulating blasts and/or M4 or M5 French-American-British [FAB] morphology). In the latter situation, it is important to initiate immediate chemotherapy. Leukapheresis for severe leukocytosis and/or leukostasis should also be considered (15). In patients with low presenting WBC count, several studies have demonstrated that initiation of therapy can delayed for several days until all the necessary diagnostic information is available (16).
At University of Texas MD Anderson Cancer Center (MDACC), most patients are enrolled on clinical trials, if eligible. The therapy is tailored to the individual patient characteristics including their cytogenetic and molecular profile. We discuss here the therapies for the various patient groups with AML.
INDUCTION THERAPY IN ACUTE MYELOID LEUKEMIA
Conventional treatment for AML, divided into remission induction and postremission therapy, has been with combinations of anthracyclines and cytarabine (ara-C).
At MDACC, young patients with AML (<60 years old) are treated with idarubicin (IDA) plus cytarabine-based regimens. The dose of IDA is 12 mg/m2 for 3 days, and ara-C is given as a continuous infusion at a dose of 1.5 g/m2 for 4 days. An alternative used by many cooperative groups is the “3+7 regimen,” where the anthracycline (ie, IDA or daunorubicin [DNR]) is usually given daily for 3 days and ara-C is given at 100 to 200 mg/m2 daily for 7 days by continuous infusion.
In clinical practice, a bone marrow aspirate is usually obtained 2 to 3 weeks after beginning therapy. A biopsy is needed only if the quality of the aspirate does not permit determination of cellularity. If the day 21 marrow is hypoplastic, therapy is usually delayed until it is clear that leukemia has reappeared, at which time the second course begins. A second repeated course of therapy can produce remissions, but these are usually of shorter duration than remissions produced after one course of therapy. The timing of a second course with persistent AML is controversial. Several cooperative groups advocate starting a second course if there is persistent AML on days 10 to 15 of chemotherapy. With high-dose ara-C (HDAC), a delay of a second course with persistent disease on days 21 to 28 may be indicated if the blasts are decreasing because most (90%) CRs are obtained after the first course and response to a second course is poor. It is important to recognize that the initial marrow obtained after a period of hypoplasia may demonstrate up to 30% to 50% blasts as a reflection of the regeneration of normal, not “leukemic,” marrow recovery. In this circumstance, follow-up (eg, at 1- to 2-week intervals) marrows may show reduction in blast percentages concomitant with a rise in neutrophils and platelets.
Typically, once in remission after treatment with the induction course, patients receive postremission therapy, with the same drugs administered at approximately monthly intervals for 4 to 12 months.
Randomized trials have attempted to identify which anthracycline (eg, IDA, DNR, mitoxantrone [MTZ], aclarubicin) is better (17). In a three-arm randomized study comparing DNR, IDA, and MTZ as part of the induction regimen for older patients, there was no advantage for any one arm (18). In contrast, in a three-arm randomized trial conducted by the European Organization for Research and Treatment of Cancer (EORTC) and Italian Group for Hematological Diseases in Adults (GIMEMA) comparing the same three agents, the 5-year disease-free survival (DFS) and OS were significantly better for patients receiving IDA and MTZ (P = .03 and .02, respectively). The recovery time was longer with IDA and MTZ (P < .0001) (19). Gardin et al analyzed pooled data from trials conducted in AML patients age ≥50 years (20). These trials compared the efficacy of IDA versus DNR in induction and consolidation. They assessed the outcomes of these patients and showed that IDA resulted in a higher CR rate of 69% (vs 61% with DNR, P = .02) but did not lead to superior OS (median OS, 14.2 months; P = .13) (20).
Different doses of DNR have been evaluated in several trials, in addition to standard-dose ara-C (100 or 200 mg/m2 daily for 7 days). Two studies showed that using DNR 90 mg/m2 had better outcomes than DNR 45 mg/m2, regardless of age. Both studies showed higher CR rates and OS in patients receiving a higher dose of DNR, without any additional toxicity. The beneficial effect was mostly seen in patients less than 50 years old and with more favorable cytogenetics (21,22). More recently, a French group also showed that DNR 60 mg/m2 had similar relapse rate, RFS, and OS compared to DNR 90 mg/m2 (23).
Even though the studies do not show survival advantage with IDA over DNR, the CR rates are definitely higher with IDA. Hence, at MDACC, IDA is the preferred anthracycline of choice.
CPX-351 is a novel formulation for delivery of ara-C and DNR synergistically to the leukemic cells. In this formulation, ara-C and DNR are encapsulated in a 5:1 fixed molar ratio that was found to be consistently synergistic in vitro. A multicenter, open-label, phase II study was conducted across 18 centers in the United States in the first-line setting. Patients >60 years old with de novo AML were randomized to receive the CPX-351 versus the 7+3 regimen. Higher response rates were noted with CPX-351, with an overall response rate (ORR) of 66.7% (vs 57.6%, P = .06). Patients with adverse cytogenetics had an improved response with CPX-351 (77% vs 38%). Improvements in the median OS (14.7 months vs 12.9 months) and EFS (6.5 months vs 2 months) were noted in the CPX-351 cohort (24). A phase III trial is currently ongoing.
Several randomized trials have assessed the efficacy of HDAC (1-3 g/m2) versus standard-dose ara-C (SDAC) (100-200 mg/m2) for induction therapy. The Cancer and Leukemia Group B (CALGB) and the Eastern Cooperative Oncology Group (ECOG) restricted their analysis to patients in CR, whereas the Southwestern Oncology Group (SWOG) compared HDAC with SDAC during induction and randomized SDAC patients to SDAC or HDAC once the patients were in CR (25). Finally, the Australian Leukemia Study Group (ALSG) randomized patients to HDAC or SDAC during induction only (Table 2-5) (26). These trials concluded that (1) the toxicity of HDAC (eg, cerebellar) outweighs the anti-AML effect in patients >65 years; (2) patients >60 years benefit from HDAC given during induction (SWOG, ALSG), in CR (CALGB, ECOG), and perhaps both (SWOG); and (3) HDAC potentially increases the cure rates to 70% to 80% in patients with inversion 16 or t(8;21) and to 30% to 40% in patients with normal karyotype, but little, if at all, in patients with adverse karyotypes. In a meta-analysis of three trials in 1,691 patients, induction with HDAC was compared to SDAC. Although there was no difference in CR rates, the 4-year RFS (P = .03), 4-year OS (P = .0005), and 5-year EFS (P < .0001) were better with HDAC (27).
Study | HDAC During: | No. of Patients | Beneficial Effect of HDAC |
---|---|---|---|
ALSG | Induction | 279 | CR duration |
SWOG | Induction and/or consolidation | 723 | Event-free survival |
ECOG | Consolidation | 170 | If age <60 years |
CALGB | Consolidation | 596 | If age <60 years |
Gemtuzumab ozogamicin (GO) is a CD33-targeted immunoconjugate linking an anti-CD33 antibody to calicheamicin. It received an accelerated approval by the US Food and Drug Administration for use in relapsed/refractory elderly AML patients but was withdrawn voluntarily by the manufacturer from the market in 2010 due to toxicity concerns. However, in a recent trial by the French Leukemia Association, patients age 50 to 70 years with previously untreated de novo AML were randomized to receive standard chemotherapy with 7+3 (DNR, ara-C) with or without GO. Gemtuzumab was administered in fractionated doses. The ORR was 78%, with 73% achieving CR and 5% achieving CR with incomplete platelet recovery (CRp). The OS (34 months vs 19 months, P = .036), EFS (15.6 months vs 9.7 months, P = .0003), and RFS (28 months vs 11 months, P = .0003) were significantly better in the GO group. Another study by the Medical Research Council (MRC) in the United Kingdom showed a benefit for the addition of GO to ara-C and anthracycline-based induction regimens (28). A recent meta-analysis examined five randomized trials in untreated patients with AML and demonstrated a benefit of GO in the frontline therapy of some subsets of patients with AML. The available data suggest utility of GO in patients with AML allowing the argument for the reconsideration of its approval in the United States.
TREATMENT OF YOUNGER PATIENTS WITH ACUTE MYELOID LEUKEMIA
Clofarabine is a second-generation nucleoside analogue that has activity in adult patients with AML. A phase I trial of clofarabine, conducted at MDACC, in combination with IDA alone versus IDA and ara-C (CIA) in patients with relapsed, refractory AML showed a CR rate of 13% versus 48%, respectively. The median duration of remission was also longer with the CIA combination (15 months) as compared to clofarabine and IDA (4.5 months) (29). This was followed by a phase II trial that investigated the CIA regimen in patients ≤60 years old with newly diagnosed AML. Patients who achieved a response (CR or CRp) went on to receive up to six cycles of consolidation therapy. The cycles were administered every 4 to 6 weeks. All patients received prophylactic antibiotics, antifungals, and antivirals. The median age of the patients was 48 years (range, 19-60 years), 66% had intermediate-risk cytogenetics, and 36% had diploid and 34% adverse karyotype. The ORR was 79%, with 74% CR and 3% CRp. Eighteen percent of patients received two induction cycles to achieve a CR/CRp, and 42% went on to receive an allogeneic stem cell transplantation (SCT) in first CR. The median OS and RFS were not reached, whereas the median EFS was 13.5 months. A subset analysis showed better OS (P = .04) and EFS (P = .04) in patients ≤40 years old as compared to >40 years old (30).
The addition of purine analogues, cladribine and fludarabine, to anthracyclines and ara-C has been associated with improved outcomes in a number of studies.
Holowiecki et al conducted a randomized phase III trial evaluating the addition of cladribine or fludarabine to DNR/ara-C in younger patients with untreated AML. Six hundred fifty-two patients, with a median age of 47 years (range, 17-60 years), were randomized to receive DNR plus ara-C (DA), DA plus cladribine (DAC), and DA plus fludarabine (DAF). The consolidation regimen was the same for all arms and included two consecutive courses of ara-C (1.5 g/m2 intravenously [IV] days 1-3) plus MTZ (10 mg/m2 IV days 3-5) and HDAC (2 g/m2 IV twice a day on days 1, 3, and5). Overall CR rate was 61%, with 56% achieving a CR after one cycle of induction and 5% after two cycles. The CR rate was higher in the DAC arm compared to the DA arm (62% vs 51%, P = .02). The CR rates were similar in the DA and DAF arms. The median OS was significantly higher in the DAC arm (24 months) compared to the DA arm (14 months, P = .02). There was no significant difference in the median OS between the DAF and DA arms (31).
Vorinostat is an oral histone deacetylase inhibitor that has been shown to have single-agent activity in AML (32). It was studied in combination with the IDA plus ara-C regimen at MDACC in a phase II trial in the frontline setting in patients with AML or intermediate-2/high-risk MDS. Induction was given as vorinostat 500 mg orally three times a day (days 1-3) along with IDA (12 mg/m2 days 4-6) and ara-C (1.5 mg/m2 continuous infusion days 4-7). Subsequently, patients achieving a remission could be treated with five cycles of consolidation and up to 12 months of single-agent vorinostat for maintenance. At a median follow-up of 82 weeks, the median OS was 82 weeks (range, 3-134 weeks) and median EFS was 47 weeks (range, 3-134 weeks). There was trend for a longer survival in the FLT3-ITD–mutated patients (91 weeks; range 6-134 weeks). The overall remission rate was 85%, with 76% achieving a CR and 9% achieving a CRp; 25% of the patients went on to undergo an allogeneic SCT in first CR.
TREATMENT OF PATIENTS ≥60 YEARS OLD
Acute myeloid leukemia in older adults (≥60 years) is considered a biologically and clinically distinct entity. The outcomes of older AML patients are poor with the standard anti-AML therapies. The analysis of the Swedish Acute Leukemia Registry (1976-2005) showed that the early death rates in all AML patients, regardless of age, were lower with intensive therapy compared to palliative therapy (33).
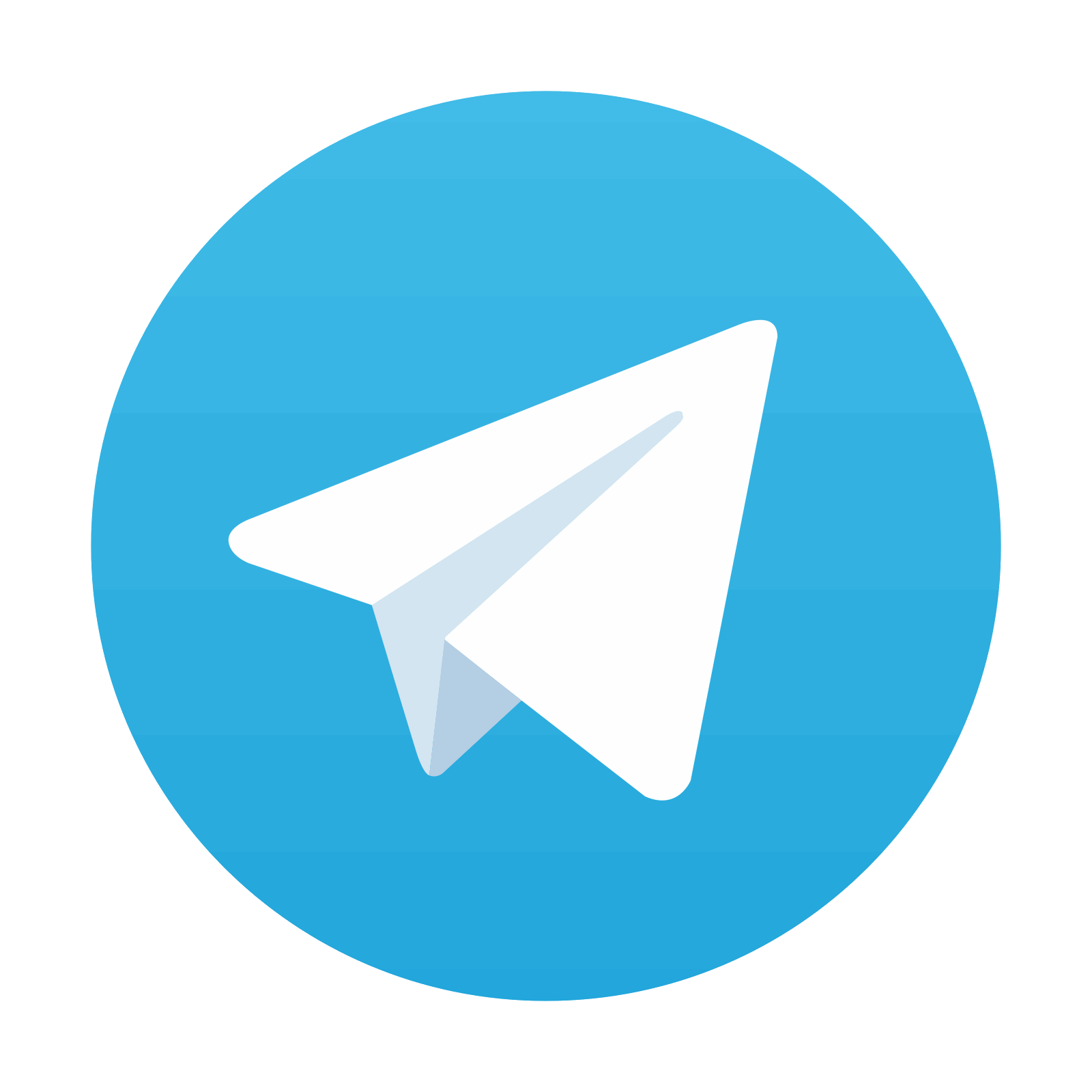
Stay updated, free articles. Join our Telegram channel

Full access? Get Clinical Tree
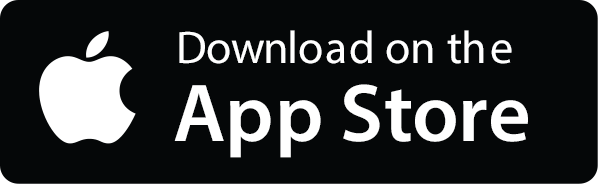
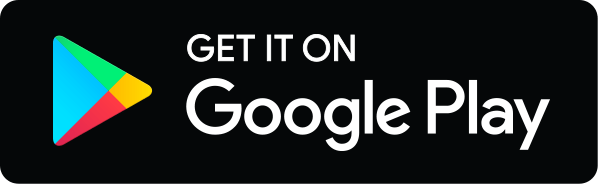