Fig. 1.1
Concentric layers of the mouse adrenal gland. (a) Schematic of the various regions of the adrenal. (b) A representative H&E longitudinal mouse adrenal section. Zones are identified by white dashed lines. c capsule, zG zona glomerulosa, zF zona fasciculata, zX X-zone, m medulla
Embryonic Adrenal Development
Adrenal embryonic development has been extensively studied [8]. In the mouse, development begins on embryonic day 9 (E9.0), or around 28 days post coitum (28 dpc) in the human, when cells in the coelomic epithelium first express the master transcriptional regulator steroidogenic factor 1 (SF1, also known as NR5A1 and AD4BP), which results in the emergence of the adrenogonadal lineage. SF1+ cells then delaminate into the adjacent mesenchyme giving rise to the adrenogonadal primordium (AGP). AGP cells, marked by expression of the Sf1-fetal adrenal enhancer (FAdE), then give rise to the fetal adrenal anlagen around E10.5 (~33 dpc). Next, cells from the neural crest invade the fetal adrenal ~E12.5 (~48 dpc), which go on to form the adrenal medulla. Subsequently, ~E14.5 (~56 dpc) the fetal cortex slowly begins to regress, while the definitive (adult) cortex emerges beneath the newly formed capsule, though distinct zones are not yet formed (Fig. 1.2). Establishing a connection between the definitive cortex and the fetal cortex, elegant lineage-tracing studies have demonstrated that the definitive cortex is indeed a direct descendent of the fetal cortex [9, 10].
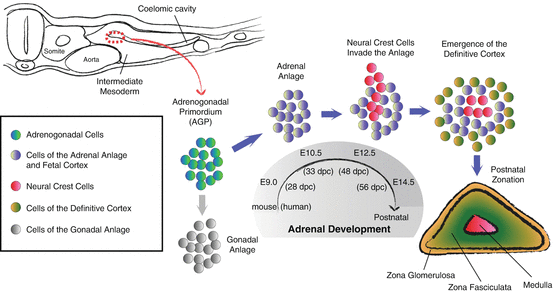
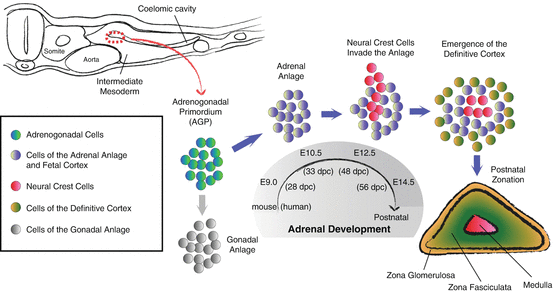
Fig. 1.2
Embryonic adrenal development in the mouse. Schematic illustration of the cellular changes during mouse and human adrenal development. The adrenogonadal primordium (AGP) originates from a thickening of the coelomic epithelium designated by the red dashed circle around E9.0 (28 dpc). At E10.5 (33 dpc), the adrenal anlage separates from the AGP and is then invaded by neural crest cells, precursors of the medullary chromaffin cells around E12.5 (48 dpc). From E14.5 (56 dpc) onward, the fetal cortical cells are slowly replaced by the definitive cortex, which gives rise to functional zones around the time of birth. Once formed, the zones are maintained throughout life
Postnatal Adrenal Development
In contrast to early embryonic development, the mechanisms underlying postnatal adrenal development , which lead to the formation and maintenance of the adrenal’s distinct zones, remain poorly understood. Detailed knowledge of how these mechanisms mediate zonation has important implications for understanding normal homeostatic functions as well as the pathological conditions that arise within the adrenal cortex. For example, it is known that control of steroidogenic output is dependent, in part, on proper maintenance of zonation over time [1]. Consistent with this, impaired zonation has been implicated in a range of conditions, including primary aldosteronism, cortisol-producing adenomas, primary pigmented nodular adrenocortical disease (PPNAD), congenital adrenal hyper- and hypoplasia, and adrenocortical carcinoma [11]. While the precise mechanisms underlying each of these conditions remain to be fully characterized, recent advances in our understanding of the cellular and molecular mechanisms underlying normal tissue homeostasis have made it possible to begin to explore key structure/function relationships within this tissue.
Adrenal Morphology
The adrenal cortex is an epithelial tissue circumscribed by a mesenchymal capsule (Fig. 1.1). The cells of the zG are organized in distinct morphological clusters, known as glomeruli, a highly conserved structure [12], which is surrounded by a basement membrane and a fenestrated capillary network [13]. zG cells are densely packed and contain scant cytoplasm, abundant rough endoplasmic reticulum, and a small number of lipid droplets and mitochondria [12, 14, 15]. In contrast, zF cells are arrayed in cord-like structures and exhibit distinctly different morphological features. zF cells are larger and more loosely packed than zG cells and contain extensive smooth endoplasmic reticulum, large gap junctions, numerous lipid droplets, and mitochondria characterized by tubulovesicular cristae [12, 14]. Also, like in the zG, zF cells are surrounded by a basement membrane and a rich capillary network. While the cells in the zR are morphologically similar to zF cells, they contain fewer lipid droplets with additional lysosomes and lipofuscin pigment granules [16]. In mice, X-zone cells are smaller than zF cells, contain an eosinophilic cytoplasm, and demonstrate a range of mitochondrial shapes with tubular cristae [6, 17].
Signaling Pathways and Zonation
The presence of morphologically distinct, yet physically contiguous, adrenocortical zones suggests tight regulation of each zone’s identity, relative size, and overall function. Recent advances in our understanding of how angiotensin II (AngII), potassium ions (K+), and adrenocorticotropic hormone (ACTH) regulate adrenal homeostasis may ultimately provide key insights into the origins of adrenal zonation and the dynamic regulation of these zones that occurs in response to physiological cues [18–27]. It is likely that multiple signaling pathways also contribute to adrenal zonation. Considerable progress has been made regarding the role of the canonical Wnt/β-catenin signaling pathway and the role of the ACTH/cyclic adenosine monophosphate (cAMP) pathway in setting the morphological and functional boundaries between the zones [11, 18, 21, 25, 28–34].
The canonical Wnt signaling pathway is active in the outer region of the cortex, overlapping with the morphological zG, and drives a transcriptional program that facilitates the production of the mineralocorticoid aldosterone [11, 29, 32]. Consistent with this, in vitro and in vivo experiments demonstrate that constitutive activation of the canonical Wnt pathway leads to an upregulation of aldosterone biosynthesis and an expansion of the morphological zG, while inhibition of the pathway leads to inactivation of aldosterone biosynthesis and contraction of the zG [28–32, 34, 35]. In contrast, the ACTH/cAMP signaling pathway is dominant in the zF and mediates the downstream transcriptional effects of ACTH on the synthesis and secretion of glucocorticoids [36–38]. Additionally, recent evidence suggests a reciprocal inhibitory effect of these two pathways, whereby Wnt signaling maintains zG zonal identity and size and also serves to inhibit expression of the zF program [30, 34]. Critical mediators of these effects include two key Wnt pathway ligands: Rspo3 (secreted from the capsule) and Wnt4 (expressed in the zG). Consistent with this, ectopic activation of Wnt signaling inactivates the zF steroidogenic program [11, 29, 30]. On the other hand, stabilization of the ACTH/cAMP signaling pathway results in activation of the zF steroidogenic program and inhibition of the Wnt signaling pathway leading to contraction of the zG [30, 33].
The overall significance of these signaling pathways in the regulation of adrenal homeostasis and zonation is made clear by the effects of somatic gain-of-function mutations giving rise to (1) aldosterone-producing adenomas (APAs) (associated with aberrant activation of the Wnt pathway) and (2) PPNAD (arising from mutations in PRKAR1A mutations, which leads to constitutive activation of ACTH/cAMP-dependent signaling) [39, 40].
Centripetal Migration and Cortical Renewal
Once established, the zG and the zF are continuously renewed throughout life and undergo dynamic hormonal feedback regulation . Despite the functional importance of these separate layers, surprisingly little is known about the cellular mechanisms that underlie their formation and ongoing maintenance. Recently, two members of the sonic hedgehog family, GLI1 and SHH, were identified as markers for adrenal progenitor cells that reside in the capsule and subcapsular regions (adjacent to the zG), respectively [41]. Consistent with the classical model of centripetal migration [42], proposed more than 70 years ago, these progenitor cells give rise to terminally differentiated zG cells, which then migrate centripetally and are thought to undergo cell fate conversion into zF cells before undergoing apoptosis at the corticomedullary junction [43].
Generation of Cyp11b2-Cre Mice
To define the molecular and cellular mechanisms underlying adrenal lineage development, we recently targeted the Cyp11b2 (aldosterone synthase) locus in mice, to generate a knock-in/knock-out Cyp11b2-Cre allele (officially known as Cyp11b2tm1.1(cre)Brit). Combined with other strains, these mice facilitate lineage-tracing, cell fate analysis and tissue-specific knock-out studies, specifically within zG cells [20]. CYP11B2 is required for the final steps of aldosterone synthesis, and its gene expression is restricted to terminally differentiated cells in the zG [43], making it a highly specific marker for zG cells. Although given the heterogeneous nature of Cyp11b2 expression with the zG under normal conditions, it is not as sensitive as other validated zG markers (e.g., β-catenin, Dab2, Dlk1) [11, 44–47]. Importantly, mice heterozygous for the Cyp11b2-Cre allele maintain normal levels of aldosterone and plasma renin activity (PRA), essential components of the renin-angiotensin aldosterone system (RAAS), indicating normal feedback regulation is maintained. In contrast, mice homozygous for the Cyp11b2-Cre allele are aldosterone deficient and demonstrate increase levels of PRA.
Direct Cell Fate Conversion
To investigate whether zG cells undergo direct cell fate conversion to zF cells, lineage-tracing studies were performed by combining Cyp11b2-Cre mice with the Rosa26 lineage reporter strain, which expresses membrane-targeted Tomato at baseline and expresses membrane-targeted green fluorescent protein (GFP) following Cre-mediated recombination (Fig. 1.3a) [20]. These studies revealed activation of the endogenous Cyp11b2 locus around the time of birth, and GFP-marked cells were entirely restricted to the zG. During the first few weeks of postnatal development, the zG was progressively marked by GFP expression (Fig. 1.3b), which subsequently gave rise to zF cells in a radial fashion, ultimately remodeling the entire zF by ~12 weeks of age (Fig. 1.3c) [20]. zG to zF cell fate conversion also functions during adrenal regeneration following dexamethasone suppression [20]. Together, these observations establish that differentiated zG cells give rise to zF cells through a process of direct cell fate conversion during postnatal adrenocortical zonation and regeneration , consistent with the model of centripetal migration.
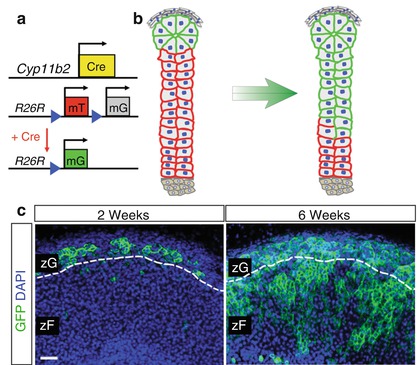
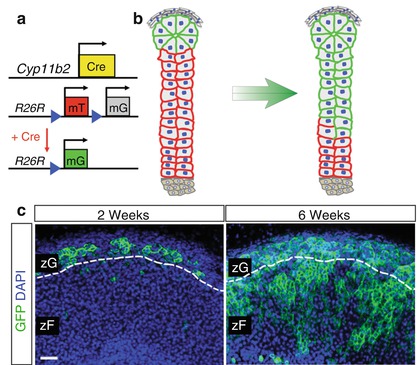
Fig. 1.3
zG cells give rise to zF cells through direct conversion . (a) Schematic illustration of the Cyp11b2-Cre and the Rosa26-mTmG allele (R26R) alleles before and after Cre-mediated LoxP recombination, which leads to deletion of mTomato and expression of mGFP. (b) Schematic illustration showing centripetal migration of GFP+ cells from the zG to the zF. (c) Representative immunofluorescent images showing centripetal migration of GFP+ cells from the zG (left, 2 weeks of age) to the zF (right, 6 weeks of age) in female mice. Scale bar, 50 μm
Role of SF1 in Cell Fate Conversion and zG Homeostasis
Understanding the mechani sms that regulate cell fate conversion has important implications for both normal and pathological states. The ability of one differentiated cell to be converted into another differentiated cell type, without passing through an undifferentiated state, has been described following the overexpression of specific transcription factors. For example, fibroblasts can be converted into myoblasts following expression of MyoD [48], and embryonic and mesenchymal stem cells can be converted into steroid-producing cells following expression of SF1 [49, 50]. The observations that SF1 plays a critical role during steroidogenic development and is sufficient to activate a steroidogenic program raised the possibility that it may play a role in cell fate conversion. Consistent with this, we observed that deletion of SF1 within zG cells prevented their conversion to zF cells (Fig. 1.4a) [20]. While the overall size of the zG remained essentially unchanged, detailed histological analysis revealed that lineage-marked zG cells had a dramatically altered cell shape, raising the possibility that these cells had undergone dedifferentiation. In addition, functional analysis revealed a state of compensated hypoaldosteronism, indicated by normal aldosterone levels and a nearly threefold increase in the levels of PRA.
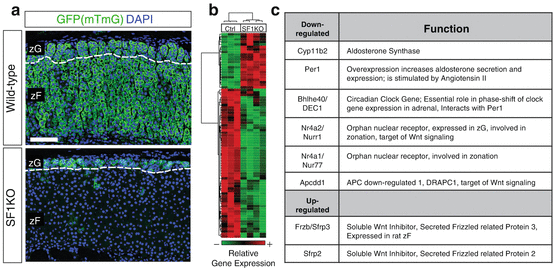
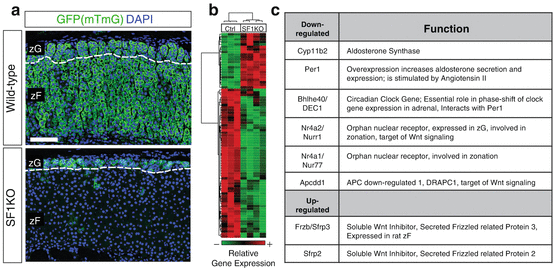
Fig. 1.4
Deletion of SF1 impairs zG-to-zF conversion and alters gene expression. (a) Representative immunofluorescent images of wild-type and SF1 KO adrenals demonstrating contribution of GFP+ cells to the zF. Note the absence of GFP+ cells in the zF in SF1 KO adrenals. Both images are t aken from 10-week-old female mice. Scale bar, 50 μm. (b) Heat map representation of differentially expressed genes from w ild-type and SF1 KO whole adrenals. Dendrogr ams represent hierarchical clustering of genes and samples. (c) Select list of genes that are down- or up-regulated in SF1 KO whole adrenals compared to wild-type whole adrenals
To identify the mechanisms by which SF1 regulates cell fate conversion and zG homeostasis, we performed transcriptome analysis comparing total RNA from SF1 KO and wild-type adrenals using Affymetrix microarray analysis. Of 35,556 probe s analyzed, 240 expressed a greater than two-fold difference in expression level and 105 of those contained unique genes (Fig. 1.4b). Among the genes showing the greatest fold changes were members of the Wnt/β-catenin signaling pathway and members of the clock gene family (Fig. 1.4c). Both of these pathways have been implicated in adrenal homeostasis, though what role they play in zG homeostasis and zonation remains largely unknown. Finally, these studies also revealed that zF cells were functionally normal, as evidenced by measurement of basal corticosterone secretion, and indicate that an “alternate (zG-independent) pathway” can contribute to zF formation. Exactly how this alternative pathway directs zF formation as well as whether it functions during normal adrenal homeostasis remains to be determined. One possibility is that when normal tissue homeostasis is severely disrupted, such as in the case of zG-specific SF1 deletion, mesenchymal cells in the capsule harboring stem-/progenitor-like potential may become activated to directly replenish the zF. Changes in gene expression identified in the microarray analysis (Fig. 1.4b) may provide new insight into these mechanisms.
Conclusions and Future Directions
In summary, the mechanisms underlying adrenocortical homeostasis and zonation during postnatal development remain largely unknown, though critical insights have recently been made. It is clear, for example, that direct conversion of zG cells into zF cells represents the major cellular mechanism by which the cortex is maintained under normal homeostatic conditions. However, it remains less clear as to the extent zG cells, alone, sustain long-term cortical renewal or to what degree zG cells rely on replenishment from the capsule, an important signaling center. Genetic lineage-tracing experiments performed by several laboratories have unequivocally demonstrated that the mesenchymal capsule can serve as a source for cellular replenishment for all steroidogenic zones as well as non-steroidogenic stromal cells [9, 41, 51]. However, an important issue raised by these studies is that constant centripetal migration of cells appears to require a much higher cellular turnover rate than provided by capsular cell activity. Hence, it is possible that differentiated cortical cells, especially the more proliferative zG population, may, in fact, play a key role in supporting the self-renewal of this tissue. Understanding which cells underlie adrenocortical self-renewal has important implications for (1) the development of future regenerative medicine strategies and for (2) understanding the pathogenesis of adrenal neoplasms. Going forward, the ability to perform “pulse-chase” lineage-tracing studies utilizing inducible mouse models will help to define the self-renewing potential of mature zG cells and to better understand the mechanisms underlying adrenal homeostasis.
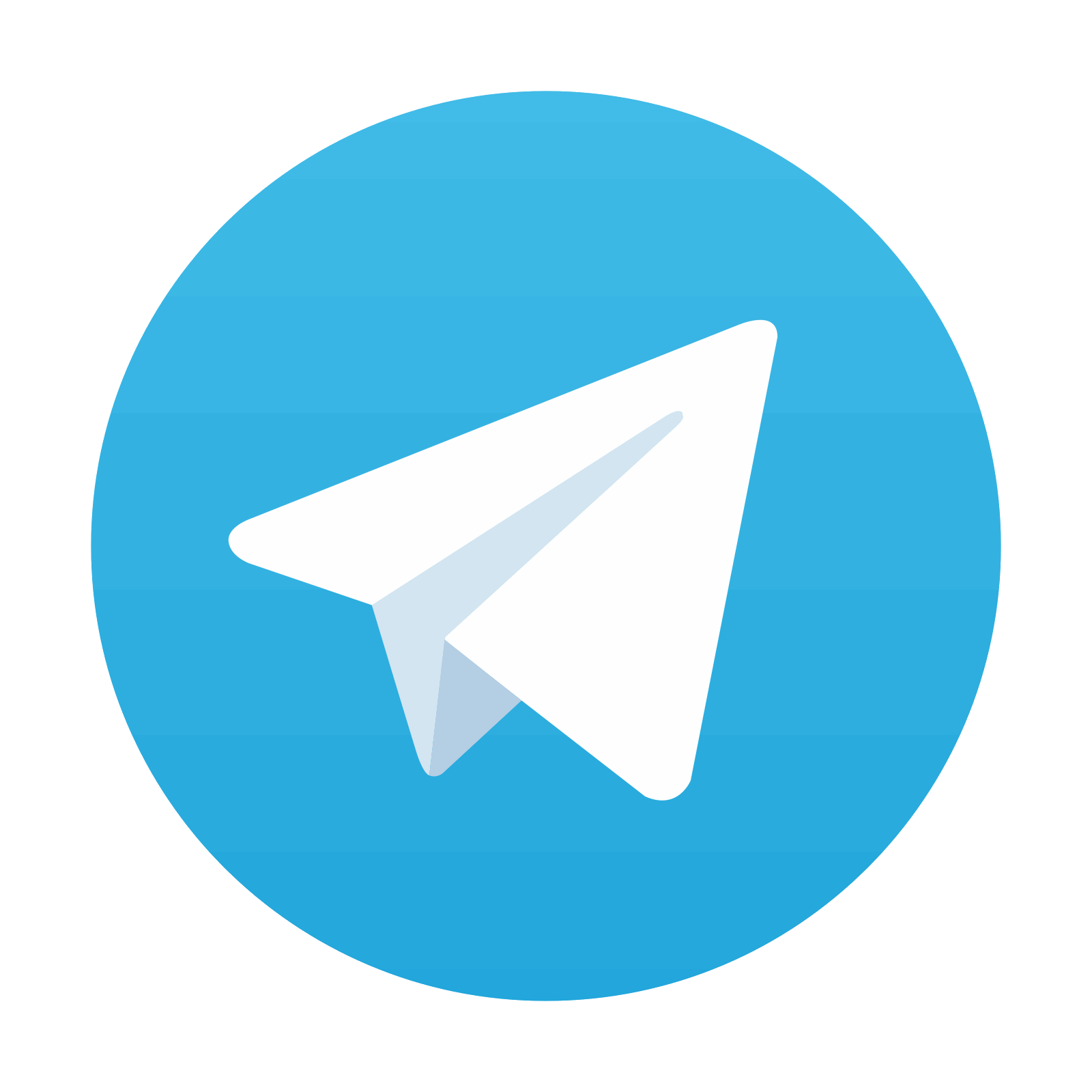
Stay updated, free articles. Join our Telegram channel

Full access? Get Clinical Tree
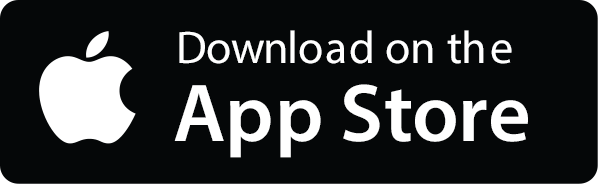
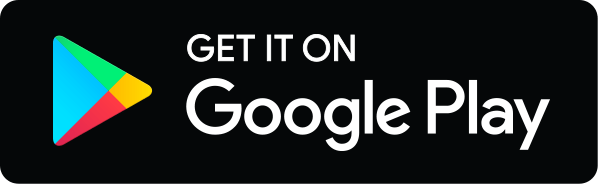