Adrenal Disorders
Dana Erickson
Steven A. De Jong
EVALUATION OF ADRENAL FUNCTION
Evaluation of Glucocorticoid Function
Cortisol
Normal production of cortisol by adrenal glands has diurnal variation. This fact, along with variations of “normal values” in multiple commercially available laboratory cortisol assays, explains the difficulties with diagnosis of adrenal pathology based on a single cortisol value. In general, a morning plasma cortisol less than 3 μg/dl is associated with high probability of adrenal insufficiency [1]. Basal serum cortisol may be used in assessment of the hypothalamic —pituitary—adrenal (HPA) axis. Meta-analysis of 12 studies showed basal cortisol less than 5 μg/dl to predict HPA axis insufficiency, values more than 13 μg/dl predicted normal function [2]. A midnight cortisol value of more than 7.5 μg/dl is useful for diagnosis of Cushing syndrome (CS) [3]. Additional limiting factors in interpretation of cortisol assays are caused by conditions that change the concentration of cortisol-binding protein: estrogen use and critical illness. In these instances, measurements of plasma free cortisol might be more appropriate [4].
Salivary cortisol reflects serum free cortisol. Various laboratory assays and collection methods reflect the need for each particular laboratory validation. This test performed late at night, late-night salivary cortisol (LNSC), appears to be particularly useful as a screening test for CS [5, 6]. For the saliva collection method, particular laboratory methods have to be taken into consideration [7]. Initial studies using salivary cortisol measurements during dynamic endocrine studies and LNSC for determination of the outcome of transsphenoidal surgery (TSS) in CS are promising [8, 9]. Salivary cortisol appeared to be helpful in the assessment of pituitary—adrenal axis in patients with cirrhosis and women on estrogens [10].
Twenty-four-hour urinary free cortisol (UFC) collection assay is the best single test for the diagnosis of CS (values at least 3 to 4 times normal are usually diagnostic
for CS). Normal values are laboratory dependent; generally, the use of immunoassays or newest high-performance liquid chromatography, or tandem mass spectrometry assays is recommended. The sensitivity and specificity depend on the cutoff value used for CS diagnosis (56%—100% sensitivity) [11]. The limitations of 24-hour collection include incomplete collection, medications that interfere with assay interpretation, periodic hypercortisolism, and pseudo-Cushing states. This test is not very helpful in the diagnosis of adrenal insufficiency. A systematic meta-analysis of diagnostic tests performed recently analyzed accuracy for initial screening tests for CS and UFC, late-night salivary cortisol, or 1-mg overnight dexamethasone suppression test (DST) and found similar likelihood ratios of these initial tests [12].
for CS). Normal values are laboratory dependent; generally, the use of immunoassays or newest high-performance liquid chromatography, or tandem mass spectrometry assays is recommended. The sensitivity and specificity depend on the cutoff value used for CS diagnosis (56%—100% sensitivity) [11]. The limitations of 24-hour collection include incomplete collection, medications that interfere with assay interpretation, periodic hypercortisolism, and pseudo-Cushing states. This test is not very helpful in the diagnosis of adrenal insufficiency. A systematic meta-analysis of diagnostic tests performed recently analyzed accuracy for initial screening tests for CS and UFC, late-night salivary cortisol, or 1-mg overnight dexamethasone suppression test (DST) and found similar likelihood ratios of these initial tests [12].
Adrenocorticotropic Hormone
Measurement of adrenocorticotropic hormone (ACTH) in plasma is important in the diagnosis of both adrenal insufficiency and CS. Two-site immunoradiometric assays should be generally obtained (along with serum cortisol values), and samples must be handled rapidly and precisely because of the instability of ACTH at room temperature. In general, levels of ACTH are elevated in primary adrenal insufficiency (PAI) and low or low normal in secondary adrenal insufficiency (SAI). In ACTH-dependent CS, ACTH is typically more than 20 pg/ml; in ACTH-independent CS, typically, it is below the low-normal limit of assay. Values in between need repeated testing or further dynamic evaluation.
Dynamic Adrenal Tests
Dynamic adrenal tests for glucocorticoid disorders are described under each pathologic condition (vide infra).
Evaluation of Mineralocorticoid Function
Resistant or multidrug hypertension, hypertension in a young patient, as well as spontaneous hypokalemia in a patient should raise the suspicion for primary hyperaldosteronism. Screening with simultaneous random plasma aldosterone concentration (PAC) to plasma renin activity (PRA) ratio (preferably in the morning after being upright for several hours) showing a value above 20 to 40 suggests positive screening test for primary hyperaldosteronism. Recent meta-analysis, however, raised uncertainty about the screening test characteristics and described lack of standardization [13]. Validated assays for plasma renin and aldosterone have to be used (various centers report a different positive cutoff for positive screening ratios), and medications that severely affect the ratio of measured hormones should be withdrawn. A multicenter study from Italy analyzing PAC/PRA ratios performed under different conditions showed good within-patient reproducibility [14]. A confirmatory test with sodium loading (NaCl tablet, typically 1 g tid × 3 days, or 3 days high-salt diet) and 24-hour urinary collection for aldosterone should follow (urinary aldosterone >14 μg/24 hr has sensitivity of 96% and specificity of 93% for diagnosis of hyperaldosteronism) [15]. A saline infusion test is used very rarely, and a cutoff value of 7 ng/dl for serum aldosterone at the end of infusion gave 100% specificity for diagnosis of primary hyperaldosteronism [16]. Mineralocorticoid deficiency is suspected when hyperkalemia and hyponatremia are present in appropriate clinical situations. No dynamic evaluation is recommended for mineralocorticoid deficiency.
Evaluation of Adrenal Medulla
Measurements of catecholamines and their metabolites are essential in the diagnostic evaluation of pheochromocytoma. For physiology and metabolism details, see the pheochromocytoma subchapter.
The utility of various available biochemical tests has been evaluated in various studies. The results of these tests depend on the type of assay used, proficiency, and lack of analytic interference of the particular laboratory and especially normative values for a given reference population. Plasma free fractionated metanephrines have the highest sensitivity (96%—99%) but a lower specificity (85%—89%), depending on the cutoffs a particular laboratory or study uses [17, 18]. Severe stress and various medications could cause false-positive values, but dietary consumption of catecholamine-rich food products did not seem to influence results of measurements of free metabolites compared to deconjugated metabolites [19]. Total urinary metanephrines combined with fractionated free urinary catecholamines have also been reported to have a relatively high sensitivity and excellent specificity for catecholamine tumor diagnosis [20]. The threshold for positive plasma metanephrine test plays a significant role in diagnostic accuracy [21]. Urinary fractionated metanephrine use is limited by lack of specificity (69%), whereas urinary vanillylmandelic acid (VMA) assays lack sensitivity (65%) [17]. Plasma catecholamine use is limited by multiple compounding factors.
ADRENAL IMAGING
Imaging studies of the adrenal gland have undergone significant advancement over the past several years. While computed tomography (CT) scanning of the adrenal gland has often been the only imaging study needed in the evaluation of a patient with adrenal tumor, there are many other radiologic tools that often provide useful information to identify hyperfunctioning, metastatic, infiltrative, infectious, incidental, or primary malignant adrenal pathology. The abundant perinephric fat, as often seen in patients with CS, allows excellent visualization of the gland. Although clinical patient information and biochemical testing are the foundation of adrenal evaluation, imaging studies can confirm, visualize, and characterize adrenal pathology to direct medical or surgical intervention [25, 26, 27]. In addition, the widespread use of cross-sectional imaging has increased the detection of incidental adrenal masses, now seen in 5% to 8% of abdominal CT imaging [28]. Clinical decisions and treatment plans frequently depend on the accuracy of these imaging studies to demonstrate and distinguish benign, malignant, and metastatic features in these adrenal tumors [29].
Computed Tomography Scanning
Despite their small size, the adrenal glands are visualized in nearly 100% of patients with CT scanning. The V-shaped right adrenal gland is usually seen directly posterior to the inferior vena cava (IVC) and measures approximately 1 × 2 × 0.5 cm. This gland is nearly the same width as the diaphragmatic stripe. The left adrenal gland, which has a similar size and shape, is located anterior to the upper portion of the kidney and directly adjacent to the aorta. Adrenal CT scanning remains an excellent initial study in the patient with adrenal disease, and 1 cm contiguous scans are routinely used to demonstrate the location, size, and tissue characteristics of most adrenal masses. This technique can also identify lymphadenopathy, obvious malignancy, and local invasion or distant metastasis associated with adrenal tumors [30]. Thinner scans using 3- to 5-mm slices are often necessary to detect and evaluate smaller functional tumors such as
aldosteronomas. Most pheochromocytomas or adrenal adenomas causing CS are 2 to 5 cm in diameter, whereas most aldosteronomas are 8 mm to 2 cm in size. The main limitation of CT scanning is its periodic inability to separate benign from malignant and functional from nonfunctional tumors of the adrenal. Cysts and myelolipomas are the only benign conditions readily identified with CT scanning. An adrenal lesion with a large amount of macroscopic fat is readily identified as a myelolipoma. Most smooth-walled water density adrenal lesions are cysts, but necrotic metastatic adrenal tumors can appear cystic and have higher density [27]. Benign adrenal adenomas are low density, lipid rich, and have an attenuation value of less than 10 Hounsfield units (HU) measured with the region of interest on noncontrast CT images (NCCT) [27]. For adrenal lesions with density greater than 10 HU, the use of IV contrast improves this distinction and benign adrenal adenomas often exhibit more than an absolute washout percentage of 60% measured 15 minutes after initial enhancement using standard contrast-enhanced CT (CECT) imaging of the adrenal gland [31]. A relative washout percentage, calculated when NCCT density is not available, of over 40% is consistent with benign adrenal disease [32]. Although contrast enhancement may increase accuracy, patients with suspected pheochromocytoma should undergo α-adrenergic blockade before contrast administration to eliminate the small risk of contrast agents precipitating a hypertensive crisis. Comparison to prior adrenal CT imaging remains important, as the volume and dimensions of benign adenomas are usually stable and only enlarge very gradually, if at all. Significant growth is frequently associated with malignancy, although adrenal hemorrhage can cause rapid enlargement in benign adrenal tumors [29]. Many surveillance imaging schedules exist for incidentally detected presumed benign adrenal tumors. While some believe this may be excessive, a reliable recommendation is for 6-month, 1-, 2-, and 3-year follow-up cross-sectional imaging to confirm lesion stability and exclude adrenal cortical malignancy. Primary adrenal malignancy should be considered in large adrenal masses with calcification, necrosis, or hemorrhage. CT of the adrenal gland has reported sensitivity, specificity, and accuracy rates of 84%, 98%, and 90%, respectively [4, 11]. Percutaneous biopsy of the adrenal gland is rarely indicated but can be safely performed by using CT guidance techniques. The left adrenal gland is usually more difficult to access for biopsy with this technique because of its posterior location [33].
aldosteronomas. Most pheochromocytomas or adrenal adenomas causing CS are 2 to 5 cm in diameter, whereas most aldosteronomas are 8 mm to 2 cm in size. The main limitation of CT scanning is its periodic inability to separate benign from malignant and functional from nonfunctional tumors of the adrenal. Cysts and myelolipomas are the only benign conditions readily identified with CT scanning. An adrenal lesion with a large amount of macroscopic fat is readily identified as a myelolipoma. Most smooth-walled water density adrenal lesions are cysts, but necrotic metastatic adrenal tumors can appear cystic and have higher density [27]. Benign adrenal adenomas are low density, lipid rich, and have an attenuation value of less than 10 Hounsfield units (HU) measured with the region of interest on noncontrast CT images (NCCT) [27]. For adrenal lesions with density greater than 10 HU, the use of IV contrast improves this distinction and benign adrenal adenomas often exhibit more than an absolute washout percentage of 60% measured 15 minutes after initial enhancement using standard contrast-enhanced CT (CECT) imaging of the adrenal gland [31]. A relative washout percentage, calculated when NCCT density is not available, of over 40% is consistent with benign adrenal disease [32]. Although contrast enhancement may increase accuracy, patients with suspected pheochromocytoma should undergo α-adrenergic blockade before contrast administration to eliminate the small risk of contrast agents precipitating a hypertensive crisis. Comparison to prior adrenal CT imaging remains important, as the volume and dimensions of benign adenomas are usually stable and only enlarge very gradually, if at all. Significant growth is frequently associated with malignancy, although adrenal hemorrhage can cause rapid enlargement in benign adrenal tumors [29]. Many surveillance imaging schedules exist for incidentally detected presumed benign adrenal tumors. While some believe this may be excessive, a reliable recommendation is for 6-month, 1-, 2-, and 3-year follow-up cross-sectional imaging to confirm lesion stability and exclude adrenal cortical malignancy. Primary adrenal malignancy should be considered in large adrenal masses with calcification, necrosis, or hemorrhage. CT of the adrenal gland has reported sensitivity, specificity, and accuracy rates of 84%, 98%, and 90%, respectively [4, 11]. Percutaneous biopsy of the adrenal gland is rarely indicated but can be safely performed by using CT guidance techniques. The left adrenal gland is usually more difficult to access for biopsy with this technique because of its posterior location [33].
Magnetic Resonance Imaging
Magnetic resonance imaging (MRI) is gaining widespread acceptance as the initial study in the evaluation of adrenal masses because of its superb soft tissue contrast, natural enhancement of certain pathologic adrenal masses, and the lack of ionizing radiation. MRI characterizes adrenal tumors by their signal characteristics on different pulse sequences and by their enhancement characteristics. It also allows multiplanar imaging to depict the relation between the adrenal mass and the kidney [34]. MRI accurately identifies benign adrenal adenomas, myelolipomas, adrenal cysts, malignant or metastatic adrenal tumors, and pheochromocytomas and generally augments information obtained with CT [35]. MRI reliably distinguishes between adenoma and metastasis in most cases and allows accurate oncologic staging, limited adrenal biopsies, and initiation of appropriate treatment regimens in the affected patient population. Chemical-shift techniques using breath-hold T1-weighted gradient echo (GRE) images can rapidly and reliably identify benign adrenal adenomas through the accurate detection of their characteristic high intracellular lipid content [34, 36]. Chemical-shift MRI offers little advantage over NCCT, but some continue to recommend this technique to differentiate lipid-rich benign adenomas from their
lipid-poor indeterminate or malignant counterparts [27]. Normal adrenal glands and adrenal tumors larger than 1 cm in diameter can be visualized in more than 90% of patients with these images, but MRI is not able to distinguish functional from nonfunctional cortical adenomas of the adrenal. T2-weighted images, however, can demonstrate unique signal intensities. Hyperintense T2-weighted images suggest the presence of pheochromocytoma, and MRI can also be useful in locating extra-adrenal pheochromocytomas. The MRI signal intensity of adrenal cortical carcinomas is variable, and they generally are heterogeneous on T1- and T2-weighted images. These tumors often appear as large, necrotic, poorly marginated retroperitoneal masses of uncertain origin where MRI fails to identify a normal ipsilateral adrenal gland [37]. These aggressive neoplasms often invade into the renal vein or IVC, and this venous extension can also be demonstrated on MRI. MRI is also an attractive imaging modality for children and pregnant patients with adrenal disease because of its lack of ionizing radiation.
lipid-poor indeterminate or malignant counterparts [27]. Normal adrenal glands and adrenal tumors larger than 1 cm in diameter can be visualized in more than 90% of patients with these images, but MRI is not able to distinguish functional from nonfunctional cortical adenomas of the adrenal. T2-weighted images, however, can demonstrate unique signal intensities. Hyperintense T2-weighted images suggest the presence of pheochromocytoma, and MRI can also be useful in locating extra-adrenal pheochromocytomas. The MRI signal intensity of adrenal cortical carcinomas is variable, and they generally are heterogeneous on T1- and T2-weighted images. These tumors often appear as large, necrotic, poorly marginated retroperitoneal masses of uncertain origin where MRI fails to identify a normal ipsilateral adrenal gland [37]. These aggressive neoplasms often invade into the renal vein or IVC, and this venous extension can also be demonstrated on MRI. MRI is also an attractive imaging modality for children and pregnant patients with adrenal disease because of its lack of ionizing radiation.
Adrenal Cortical Scintigraphy
Hormone production in the adrenal cortex begins with cholesterol, and several radiolabeled cholesterol analogs have been developed to allow localization and functional information regarding adrenal cortical tumors. The only one currently approved for use in the United States is [131I]-6β-iodomethylnorcholesterol (NP-59). Unfortunately, NP-59 scanning has been largely abandoned as it is difficult to obtain, expensive, time consuming, and involves significant radiation exposure to the patient. The material, however, is safe to administer, and the accuracy rate of NP-59 to identify and distinguish correctly a Cushing adrenal adenoma or an aldosteronoma from bilateral adrenal hyperplasia approaches 90% [38]. Detection of aldosteronomas with NP-59 requires discontinuation of diuretic and antihypertensive medication, and accuracy rates are increased if the patients are pretreated with dexamethasone.
Adrenal Medullary Scintigraphy
Metaiodobenzylguanidine (MIBG), a radiolabeled derivative of guanethidine with no pharmacologic effect, was developed in 1980. It is the most frequently used substance to image the adrenal medulla and other sympathetic tissues. It is incorporated into sympathetic nerve cells as a catecholamine precursor. [131I] MIBG is the most commonly used isotope, but recent studies suggest that123I is more accurate with less radiation exposure to the patient. Single-photon emission computed tomography technology may also improve the sensitivity and accuracy of this imaging tool. MIBG scanning is diagnostic for neoplasms producing excess catecholamine and has successfully detected pheochromocytomas, neuroblastomas, and other neuroendocrine tumors such as paragangliomas, carcinoid tumors, and medullary carcinomas of the thyroid. The most common role for MIBG is in patients with a biochemical diagnosis of pheochromocytoma and normal conventional adrenal imaging studies. MIBG can identify paragangliomas and metastatic pheochromocytomas to guide further imaging. The sensitivity and specificity of MIBG scanning for pheochromocytoma and neuroblastoma are between 80% and 100%. An alternative to MIBG is radiolabeled octreotide [111I(indium)]-DTPA (diethylenetriaminepenta-acetate) octreotide, which can detect paragangliomas and neuroendocrine tumors containing cell-surface receptors for somatostatin [39]. Unfortunately, this imaging modality is only 30% sensitive [40]. The use of MIBG may be decreasing with the adoption of positron emission tomography (PET) scanning, which often demonstrates increased FDG activity [41].
Positron Emission Tomography
Several different radiopharmaceuticals can detect increased cellular activity in hyperfunctional adrenal states more specifically than does conventional imaging. These agents can also target specific adrenal enzymes that are expressed in adrenal tumor cells. As an example, [18F]fluorodopamine PET scanning allows tumor visualization within minutes of injection of the imaging agent, and spatial resolution is excellent. Malignant cells are frequently glucose avid, and PET scanning with the glucose analog18F-fluoro-deoxyglucose (18F FDG) is effective in differentiating benign from malignant and metastatic adrenal lesions with 97% sensitivity and 91% specificity [42, 43]. PET scanning also correctly localizes adrenal and extra-adrenal pheochromocytomas that lack the ability to concentrate MIBG [44, 45]. PET imaging is now frequently combined with CT imaging (CT/PET) to assess both the gross appearance of the lesion and its metabolic activity [27].
Adrenal Vein Sampling
Adrenal vein sampling is useful in patients with functional tumors of the adrenal glands such as in patients with CS or primary hyperaldosteronism. These patients have normal or equivocal findings on CT, MRI, or adrenal scintigraphy, and these imaging studies cannot distinguish between a unilateral aldosteronoma requiring adrenalectomy from bilateral adrenal hyperplasia treated medically [46]. Although the left adrenal vein is accessible in nearly all patients, the right adrenal vein can be more difficult to cannulate. Patients with primary hyperaldosteronism receive an infusion of cosyntropin, and blood from both adrenal veins and the distal IVC is measured for both aldosterone and cortisol. Adrenal vein cortisol should be five times greater than IVC cortisol to verify the position of the venous catheter in the adrenal vein. The ratios of aldosterone to cortisol for both adrenal veins are compared, and a ratio of 4:1 or greater reliably identifies a small unilateral aldosteronomas, whereas a ratio of less than 3:1 usually indicates bilateral adrenal hyperplasia [47]. Selective venous sampling in patients with CS is performed in a similar manner without the infusion of cosyntropin. Cortisol levels from both adrenal veins and the IVC are compared to distinguish between bilateral adrenal hyperplasia and adrenal adenoma in patients with CS.
PRIMARY HYPERALDOSTERONISM
Definition and Etiology
Primary hyperaldosteronism is the excessive autonomous adrenal secretion of aldosterone, resulting in elevation of PAC and suppression of PRA. While the exact cause is unknown, this syndrome results in a rare form of surgically correctable hypertension, polyuria, weakness, hypokalemia, sodium retention, and mild metabolic alkalosis. A small, benign, unilateral, aldosterone-producing adrenal tumor, first described by Jerome Conn in 1955, was historically the most common cause of primary hyperaldosteronism in 70% of patients. Currently though, the contemporary incidence of idiopathic bilateral macronodular or micronodular hyperplasia of the adrenal cortex causing primary hyperaldosteronism has increased to over 50%, largely due to widespread screening of hypertensive patient populations [48, 49]. Other far less common causes of primary hyperaldosteronism include unilateral primary adrenal hyperplasia detected by adrenal venous sampling (AVS), glucocorticoid-suppressible hyperaldosteronism, a rare familial form of hypertension that affects 1% to 3% of patients with primary hyperaldosteronism [50], and aldosterone-producing adrenal carcinoma [51].
Epidemiology
The prevalence of primary hyperaldosteronism in unselected patients with hypertension is 0.5% to 2%, but centers routinely obtaining PAC/PRA ratios detected primary hyperaldosteronism in 8% to 15% of their hypertensive patients, representing a 10-fold increase in this diagnosis [52]. Age at diagnosis ranges between 30 and 60 years, and about 1% of all adrenal masses discovered incidentally are found to be aldosterone-producing adrenal adenomas. Aldosteronomas occur more commonly in female patients, by a 2:1 ratio, and are rare in children. Patients with aldosteronomas tend to be younger, have more severe hypertension and hypokalemia, have higher urine and serum aldosterone levels, and respond better to spironolactone than patients with bilateral adrenal disease. Bilateral idiopathic adrenal hyperplasia causing primary hyperaldosteronism is more common in men and usually appears in patients at an older age when compared to aldosteronoma. High blood pressure is present, but this sign is a common medical problem and is not sufficiently specific to identify this endocrinopathy. Although hypertension in these patients is often of moderate severity, multiple medical regimens to control blood pressure are usually unsuccessful, causing the diagnosis of primary hyperaldosteronism to be considered [50].
Pathophysiology
Aldosterone is the final product in the biosynthesis of mineralocorticoids produced from the zona glomerulosa of the adrenal cortex. Factors stimulating the synthesis and release of aldosterone include angiotensin II, potassium, ACTH, and decreased renal perfusion or circulating blood volume. The clinical manifestations of primary hyperaldosteronism arise from the autonomous production of aldosterone, causing altered sodium and potassium homeostasis. Aldosterone normally enhances the reabsorption of sodium from the distal renal tubules in exchange for potassium and hydrogen excretion. Excessive aldosterone secretion leads to sodium and water retention and increased potassium and hydrogen excretion. The result is volume expansion and hypertension, suppressed PRA, hypokalemia, and metabolic alkalosis [48]. Serum sodium levels usually remain normal because of a parallel increase in the water content of the blood, but mild hypernatremia can be present. Hypokalemia appears to be responsible for most of the clinical symptoms of primary hyperaldosteronism, such as proximal muscle weakness and cramps, polyuria, polydipsia, nocturia, headache, and fatigue.
Diagnosis
Unlike most endocrine disorders, primary hyperaldosteronism does not have characteristic symptoms or signs, but this disorder should be considered in all patients with hypertension, unprovoked hypokalemia, and metabolic alkalosis. The use of antihypertensive medications that specifically block aldosterone secretion, such as spironolactone and other similar diuretics, must be stopped for 4 to 6 weeks before biochemical testing. Screening studies such as measurement of PRA, PAC, and potassium levels, along with a 24-hour urine collection for aldosterone, potassium, and sodium, should begin the evaluation. Most patients with primary hyperaldosteronism have a serum potassium level less than 4.0 mEq/l, and some can range from 2.5 to 3.5 mEq/l at the time of clinical presentation [40, 50]. Recent studies, however, describe a rising incidence of normokalemia seen in as high as two-thirds of patients with early primary hyperaldosteronism found by screening laboratory studies performed on hypertensive patients [53]. Despite hypokalemia and a total body deficit of potassium, the 24-hour urine collection for potassium demonstrates inappropriate kaliuresis. Diuretic-induced hypokalemia that does not respond to potassium replacement also suggests the presence of primary hyperaldosteronism.
Plasma aldosterone levels can be elevated or normal, but the PRA is invariably suppressed. Low PRA excludes the presence of secondary hyperaldosteronism, characterized by increased renin and aldosterone levels, and resulting from renal artery stenosis, cirrhosis, intravascular volume loss, congestive heart failure, and other disorders that decrease renal perfusion. Plasma aldosterone levels usually exceed 15 to 30 ng/l, and PRA is usually less than 1 ng/l. A ratio of plasma aldosterone to PRA that exceeds 30 to 50 is highly suggestive of excess endogenous aldosterone production [48, 50]. A PAC/PRA ratio of 20:1 along with a PAC greater than 15 ng/dl was also found to be highly sensitive in establishing the diagnosis [54].
Oral or intravenous sodium chloride administration may be necessary in some hypertensive patients where the distinction between primary and secondary hyperaldosteronism is less clear. Primary autonomous aldosterone secretion is confirmed when PAC was greater than 5 to 10 ng/dl after a 2-l saline infusion over 4 hours or urinary aldosterone levels were greater than 12 ng/dl after liberal oral intake of high-sodium-content foods over 3 to 4 days [52]. In addition, the inability to reduce plasma aldosterone levels and increase PRA after the administration of captopril, an angiotensin-converting enzyme (ACE) inhibitor, also suggests primary hyperaldosteronism [50].
After the diagnosis of primary hyperaldosteronism is confirmed, the main cause of this syndrome, either unilateral adrenal adenoma or idiopathic bilateral adrenal hyperplasia, must be determined because of significant treatment implications [55]. Postural testing can be used to make this distinction because unilateral aldosterone-producing adrenal adenomas are unresponsive to the effects of angiotensin II. Plasma levels of aldosterone are measured in patients assuming a supine position and then again after 4 hours of standing. Angiotensin II and PRA increase in the standing position, but patients with aldosterone-producing adrenal adenomas have no increase in their aldosterone levels during this test. Conversely, aldosterone levels in patients with idiopathic bilateral adrenal hyperplasia usually increase. Postural testing can correctly differentiate between unilateral and bilateral adrenal disease in 75% to 85% of patients with primary hyperaldosteronism [48, 55, 56]. CT scanning of the adrenal glands has largely replaced postural testing in many centers as the initial study of choice to identify the aldosteronoma, but postural testing is helpful in equivocal cases or when a nonfunctional adrenal adenoma is suspected. Measurement of 18-hydrocorticosterone is not uniformly available but can also distinguish adrenal adenoma from bilateral adrenal hyperplasia. Plasma levels of 18-hydrocorticosterone greater than 100 ng/dl are indicative of unilateral adrenal adenoma with a sensitivity of 60% to 85% [57], but postural testing and serum 18-hydrocorticosterone levels alone cannot lateralize the disease process or localize the aldosteronoma [58].
Imaging of the adrenal glands, after diagnostic confirmation of primary hyperaldosteronism, is best performed with enhanced high-resolution CT scanning of both adrenal glands, which has an 80% to 90% sensitivity rate in detecting aldosteronomas. This technique, however, can also detect tiny adrenal nodules or adrenal limb thickening that may or may not have any clinical significance. Aldosteronomas are usually 0.5 to 2 cm in diameter and require imaging in 3-mm increments rather than the conventional 1-cm CT sections. It is important to visualize the contralateral adrenal gland adequately because enlargement of both glands may suggest idiopathic bilateral adrenal hyperplasia. The sensitivity of MRI is equal to that of CT scanning for these patients [50].
Patients under 40 years of age with biochemically confirmed primary hyperaldosteronism and CT imaging demonstrating a solitary adrenal adenoma larger
than 1 cm with rapid contrast washout and a normal contralateral adrenal gland can undergo surgical resection with no further testing. Older surgical candidates, however, with primary hyperaldosteronism and either no adrenal mass, bilateral adrenal masses, an adrenal microadenoma, or equivocal findings on CT or MRI should undergo adrenal vein sampling for aldosterone and cortisol levels to determine the origin of the primary hyperaldosteronism. Adrenal vein sampling for aldosterone has a diagnostic sensitivity of 96% but does require significant technical expertise, as the short right adrenal vein can be challenging to cannulate. Technical failure rates range from 5% to 30%. ACTH is administered, and aldosterone and cortisol levels are measured from both adrenal veins and the IVC. An adrenal vein/IVC cortisol ratio of 5:1 ensures proper placement of the catheter into the respective adrenal vein. A unilateral aldosterone-producing adenoma (APA) produces a ratio of aldosterone to cortisol that is four to five times greater than that of the opposite side [58]. Bilateral adrenal disease reveals bilateral elevation of this ratio and no appreciable gradient. One study compared CT and adrenal vein sampling in 24 patients with primary hyperaldosteronism [59]. CT led to diagnosis of unilateral adenoma in 19 patients and hyperplasia in seven. After AVS, unilateral adenoma was diagnosed in 22 patients. Of the seven patients with bilateral nodules, AVS correctly identified the unilateral adenoma in six. Other studies [60] found that CT imaging without AVS would have inappropriately excluded 22% of the patient population from surgical consideration and exposed 25% of patients to unnecessary or inappropriate adrenalectomy.
than 1 cm with rapid contrast washout and a normal contralateral adrenal gland can undergo surgical resection with no further testing. Older surgical candidates, however, with primary hyperaldosteronism and either no adrenal mass, bilateral adrenal masses, an adrenal microadenoma, or equivocal findings on CT or MRI should undergo adrenal vein sampling for aldosterone and cortisol levels to determine the origin of the primary hyperaldosteronism. Adrenal vein sampling for aldosterone has a diagnostic sensitivity of 96% but does require significant technical expertise, as the short right adrenal vein can be challenging to cannulate. Technical failure rates range from 5% to 30%. ACTH is administered, and aldosterone and cortisol levels are measured from both adrenal veins and the IVC. An adrenal vein/IVC cortisol ratio of 5:1 ensures proper placement of the catheter into the respective adrenal vein. A unilateral aldosterone-producing adenoma (APA) produces a ratio of aldosterone to cortisol that is four to five times greater than that of the opposite side [58]. Bilateral adrenal disease reveals bilateral elevation of this ratio and no appreciable gradient. One study compared CT and adrenal vein sampling in 24 patients with primary hyperaldosteronism [59]. CT led to diagnosis of unilateral adenoma in 19 patients and hyperplasia in seven. After AVS, unilateral adenoma was diagnosed in 22 patients. Of the seven patients with bilateral nodules, AVS correctly identified the unilateral adenoma in six. Other studies [60] found that CT imaging without AVS would have inappropriately excluded 22% of the patient population from surgical consideration and exposed 25% of patients to unnecessary or inappropriate adrenalectomy.
Iodocholesterol scanning with [6β-131I]iodomethyl-19-norcholesterol (NP-59) after dexamethasone suppression is a less-used imaging study to differentiate adenoma from hyperplasia. This study has a reported sensitivity of 88% and can identify unilateral uptake in larger adrenal aldosteronomas and bilateral uptake in adrenal hyperplasia [48, 50]. In a study of 41 patients who were examined by using CT and iodocholesterol scanning with NP-59 after dexamethasone suppression, scintigraphy correctly identified bilateral and unilateral lesions in 92% of cases compared with 58% by CT [61]. Aldosterone-producing adrenal carcinoma often demonstrates no uptake on adrenal scintigraphy [51]. Unfortunately, this study depends heavily on the size of the adenoma, and its declining use is limited by the availability of the radioisotope.
Treatment
Unilateral adrenal aldosteronomas are treated with laparoscopic or open adrenalectomy [62]. Adrenal resection is performed after a 1- to 2-week course of spironolactone at 25 to 400 mg/d in divided doses to correct the hypokalemia and other associated volume and metabolic derangements. Adrenalectomy is likely to be successful if the patient’s hypertension is efficiently controlled with preoperative spironolactone [63]. The intra-abdominal or posterior retroperitoneal laparoscopic approach is the method of choice because of fewer wound complications, less patient discomfort, shorter hospitalizations, and a more rapid return to work and normal activity. Visual examination of the sectioned adrenal gland reveals a solitary bright golden yellow tumor with well-defined borders arising from the otherwise normal adrenal parenchyma. Spironolactone should be discontinued after unilateral adrenalectomy, and potassium supplements can be rapidly tapered and then stopped. Resolution of preoperative hypokalemia usually occurs within 5 to 7 days of adrenalectomy in 95% of patients, and cure of hypertension over several months is expected in 70% to 80% of patients with normal renal function [62, 64]. The remaining 20% to 30% of patients usually experience improvement in blood pressure control, and they often require
fewer antihypertensive medications at lower doses after adrenalectomy. Both recurrence of primary hyperaldosteronism incidence of adrenal insufficiency after unilateral adrenalectomy for aldosteronoma is rare.
fewer antihypertensive medications at lower doses after adrenalectomy. Both recurrence of primary hyperaldosteronism incidence of adrenal insufficiency after unilateral adrenalectomy for aldosteronoma is rare.
Hypertension and hypokalemia in patients with idiopathic bilateral adrenal hyperplasia are best treated medically with potassium-sparing diuretic agents such as spironolactone, amiloride, and other antihypertensive agents. These medications can be associated with gynecomastia, menstrual irregularities, and impotence [50], but the use of eplerenone, a more selective aldosterone antagonist, may be associated with fewer undesirable side effects [65]. Hypertension in these patients responds poorly to bilateral adrenalectomy, which invariably leads to hypocortisolism, otherwise known as Addison disease. Aldosterone-producing adrenal carcinomas occur in 1% of patients with primary hyperaldosteronism and have an overall 5-year survival rate of 35%. These tumors are often large with aggressive invasive features and require open adrenalectomy for complete resection or debulking [51].
CUSHING SYNDROME
The constellation of clinical features that result from persistent, inappropriate elevation of glucocorticoids is described as CS. The incidence of endogenous CS is low, 0.7 to 2.4 per million population; however, recent studies suggest perhaps a higher incidence in a patient population with poorly controlled diabetes or osteoporosis [66, 67] in some but not all studies [68].
Etiology and Pathogenesis
Exogenous administration of glucocorticoids (iatrogenic or factitious) is the most common cause of CS. Approximately 80% of patients with endogenous CS have ACTH-dependent disease, most frequently caused by pituitary microadenomas (i.e., Cushing disease; see Chapter 1) [69]. Overproduction of ACTH in ACTH-dependent Cushing may also be due to ectopic overproduction of ACTH or corticotropin-releasing hormone (CRH), caused by certain malignancies (Table 3.1) [70]. The excessive ACTH or CRH production leads to overproduction of cortisol by adrenal glands. In ACTH-independent CS (15%—20% of endogenous causes), autonomous increase in cortisol production by adrenal glands is found, resulting in suppression of ACTH. The etiology of this autonomous cortisol overproduction is represented by: adrenal adenomas, adrenal carcinomas, bilateral or unilateral macronodular adrenal hyperplasia, and micronodular adrenal hyperplasia. The expression of certain ectopic promiscuous receptors (gastric inhibitory polypeptide, vasopressin, β-adrenergic receptors, serotonin, and luteinizing hormone receptors) in adrenocortical cells explains some of the causes of macronodular adrenal hyperplasia [71]. Certain conditions such as affective disorders, stress, and obesity can lead to mild elevations in plasma or urinary cortisol levels, the so-called pseudo-Cushing state. The entity of subclinical CS (SCS) is described in the section on adrenal incidentalomas.
Clinical Features
The clinical manifestations of hypercortisolism are common to all forms of CS and include hypertension; central obesity; diabetes mellitus [72]; acne; androgenic hirsutism; signs of protein catabolism such as myopathy, osteopenia, or osteoporosis; cutaneous lesions (i.e., wide violaceous striae, tinea versicolor, verrucous vulgaris, ecchymosis); hyperpigmentation; and psychiatric manifestations (depression, cognition, and vegetative function). Increased thrombotic events
were well documented in recent meta-analysis [73].The classic features may not be present in ectopic ACTH production from malignancies, because of shorter duration of hypercortisolemia. Impaired quality of life is seen in patients with CS after remission of CS [74].
were well documented in recent meta-analysis [73].The classic features may not be present in ectopic ACTH production from malignancies, because of shorter duration of hypercortisolemia. Impaired quality of life is seen in patients with CS after remission of CS [74].
Table 3.1. Causes of Cushing Syndrome | ||||||||||||||||||||||||||||||||||||||||||||||||||||||||||||||||||||
---|---|---|---|---|---|---|---|---|---|---|---|---|---|---|---|---|---|---|---|---|---|---|---|---|---|---|---|---|---|---|---|---|---|---|---|---|---|---|---|---|---|---|---|---|---|---|---|---|---|---|---|---|---|---|---|---|---|---|---|---|---|---|---|---|---|---|---|---|
|
Evaluation
Diagnostic Tests
Measurement of 24-hour UFC is the most reliable screening test for CS. The sensitivity is reported to be between 56% and 100%, and the specificity varies depending on study population, assays, and laboratory cutoffs for elevation [75]. A value that is more than four times the upper limit of normal is typically considered to be indicative of CS.
Loss of circadian rhythm with high plasma cortisol level (7.5 mg/dl) between 10 p.m. and midnight is an early indicator of CS [3]. Late-night salivary cortisol testing in a total of 339 patients with CS measured by various methods, laboratories, and cutoff generation showed pooled sensitivity of 92% and specificity of 96% in a recent meta-analysis [6].
The overnight DST (dexamethasone, 1 mg PO administered at 11 p.m., followed by 8 a.m. plasma cortisol) is used as a screening test for CS [76]. Various criteria for normal suppression (traditionally <5 μg/dl or recently <1.8 μg/dl) applied to various study populations and frequently lack of matching controls influence sensitivity and specificity of the test [77]. Data regarding salivary post—1-mg dexamethasone cortisol test are very limited. A systematic meta-analysis of diagnostic tests performed recently analyzed accuracy for initial screening tests for CS and UFC, late-night salivary cortisol, or 1-mg overnight DST and found similar likelihood ratios of these initial tests [12].
In the 2-day low-dose dexamethasone test, dexamethasone, 0.5-mg tablet, is taken every 6 hours for 2 days, followed by plasma cortisol determination at 8 a.m. Normal response includes plasma cortisol level suppression to less than
5 μg/dl (or more stringent cutoff of <1.8 μg/dl) and UFC suppression below the lower limit of normal [12, 76, 77, 78]. Dexamethasone—0.5 mg every 6 hours for 2 days followed 2 hours later by intravenous ovine CRH, 1 μg/kg—yields plasma cortisol of less than 1.4 to 2.5 mg/dl at 15 minutes in patients with pseudo-Cushing [79, 80]. Various medications can confound interpretation of this test [81].
5 μg/dl (or more stringent cutoff of <1.8 μg/dl) and UFC suppression below the lower limit of normal [12, 76, 77, 78]. Dexamethasone—0.5 mg every 6 hours for 2 days followed 2 hours later by intravenous ovine CRH, 1 μg/kg—yields plasma cortisol of less than 1.4 to 2.5 mg/dl at 15 minutes in patients with pseudo-Cushing [79, 80]. Various medications can confound interpretation of this test [81].
Baseline ACTH Level
An inappropriately normal or elevated baseline plasma ACTH level (i.e., >20 pg/ml) indicates ACTH-dependent CS. Cushing disease usually appears with ACTH levels less than 200 pg/ml, whereas ACTH levels in ectopic ACTH syndromes range from 200 to 1,000 pg/ml. A low ACTH level (typically <5 pg/ml) represents ACTH-independent CS.
High-Dose Dexamethasone Suppression
The high-dose dexamethasone suppression (HDDS) test is done to differentiate between pituitary ACTH overproduction and ectopic ACTH production and occasionally in ACTH-independent CS. Dexamethasone, 2 mg orally every 6 hours for 2 days, is followed by plasma cortisol estimation at 8 a.m. Plasma cortisol below 50% of basal values or 24-hour UFC less than 90% of basal values indicates Cushing disease [82]. Absence of response to HDDS indicates ectopic ACTH or adrenal CS. In the overnight HDDS test, a single dose of 8-mg dexamethasone is given at 11 p.m., and 8 a.m. plasma cortisol is measured the next day. The test is interpreted the same way as is standard HDDS testing. In a limited series, the sensitivity and specificity of the overnight HDDS test are similar to those of the standard HDDS test; however, a higher plasma cortisol suppression (>68%) cutoff was used for the latter [83]. Effectiveness analysis of both tests points toward the limitations of these tests by using standard criteria in interpretation [84]. Note that the pretest probability of pituitary Cushing disease is high, which limits the usefulness of these tests. In general, these tests are not used very frequently in current practice.
Invasive testing including inferior petrosal or cavernous sinus sampling (highest sensitivity and specificity), as well as CRH stimulation tests, are discussed in the section on Cushing disease in Chapter 1.
Radiologic Imaging
Thin-section CT (3-mm cuts) or MRI of the adrenal glands to look for the cause of adrenal autonomy is the next step in evaluation of patients with ACTH-independent CS. Imaging of ACTH-dependent CS is discussed in Chapter 1.
Treatment
The goal of therapy is to eradicate the cause of CS. Treatment of pituitary Cushing disease is described in Chapter 1. Optimal therapy in cases of ACTH-independent CS includes surgical removal of adrenal adenoma or carcinoma. In cases of ACTH-independent bilateral hyperplasia (micronodular or macronodular), as well as in certain cases of persistent or recurrent ACTH-dependent disease, bilateral adrenalectomy is the treatment of choice. The laparoscopic approach to surgery has reduced the surgical morbidity of adrenalectomy. The hospital stay is shorter, and complications are much less frequently encountered compared with results associated with open surgery. Mortality is reported at 0.2% [85].
Medical treatment can be used when surgery is contraindicated, if the tumor is metastatic or occult, or if surgery and radiation therapy (for pituitary disease) have failed. Medical therapy may also be used to attempt to stabilize patients preoperatively.
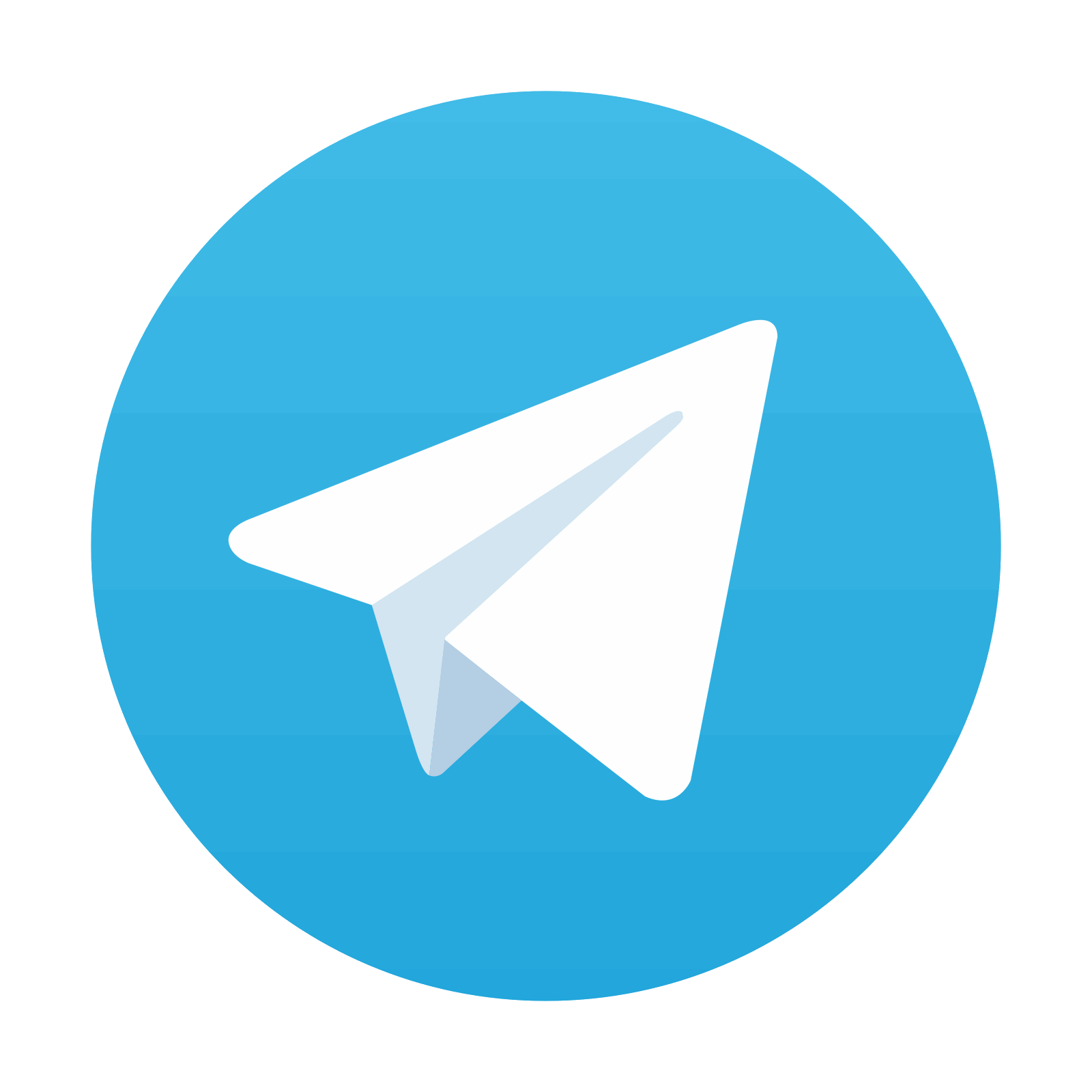
Stay updated, free articles. Join our Telegram channel

Full access? Get Clinical Tree
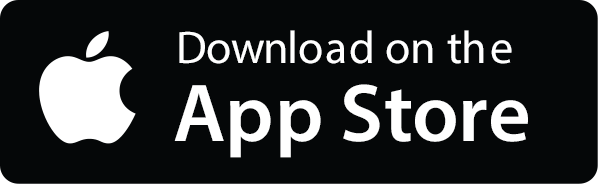
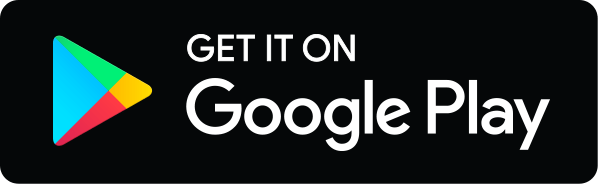
