Therapeutic exposure
Potential late effect
Vincristine, vinblastine
Peripheral neuropathy, Raynaud’s phenomenon
Corticosteroids
Cataracts, osteopenia, osteoporosis, avascular necrosis
Mercaptopurine
Hepatic dysfunction, veno-occlusive disease
Methotrexate
(Systemic) osteopenia, osteoporosis, renal dysfunction, hepatic dysfunction
(Intrathecal) neurocognitive deficits, clinical leukoencephalopathy
Cytarabine (high-dose)
Neurocognitive deficits, clinical leukoencephalopathy
Anthracyclines
Cardiomyopathy, arrhythmias, secondary AML
Alkylating agents
Hypogonadism, infertility, secondary AML/MDS
Busulfan, carmustine, lomustine
Pulmonary dysfunction, infertility
Cyclophosphamide, ifosfamide
Hemorrhagic cystitis, dysfunctional voiding, bladder malignancy, renal dysfunction (ifosfamide only)
Heavy metals (platinum)
Ototoxicity, peripheral sensory neuropathy, renal dysfunction, dyslipidemia
Etoposide, teniposide
Secondary AML
Bleomycin
Pulmonary dysfunction
Mantle radiation
Hypothyroidism, premature cardiovascular disease, cardiac valvular disease, cardiomyopathy, arrhythmias, carotid artery disease, scoliosis/kyphosis, second malignant neoplasm in radiation field (e.g., thyroid, breast), pulmonary dysfunction
Inverted Y radiation
Hypogonadism, infertility, adverse pregnancy outcome, second malignant neoplasm in radiation field (e.g., gastrointestinal)
Cranial or craniospinal radiation
Neurocognitive deficits, clinical leukoencephalopathy, cataracts, hypothyroidism, second malignant neoplasm in radiation field (e.g., skin, thyroid, brain), short stature, scoliosis/kyphosis, obesity
Splenectomy
Acute life-threatening infections
Blood products
Chronic viral hepatitis, HIV
28.2 Medical Issues
28.2.1 Late Mortality
Overall mortality among AYA cancer survivors has been described to be tenfold that of the general population [90, 93]. The CCSS assessed overall and cause-specific mortality in a retrospective cohort of 20,227 5-year survivors of childhood cancer and demonstrated a 10.8-fold excess in overall mortality [90]. Risk of death was statistically significantly higher in females, individuals diagnosed with cancer before the age of 5 years, and those with an initial diagnosis of leukemia or brain tumor. The excess mortality was due to death from primary cancer, second cancer, cardiotoxicity, and noncancer death and existed for up to 25 years after the initial cancer diagnosis. Specific data regarding late mortality in individuals first diagnosed with cancer as young adults is more limited, but a population-based study from the Finland Cancer Registry identified 16, 769 5-year survivors of early-onset cancer (age 0–34 at diagnosis, 5,352 age 0–19, and 11,417 age 20–34) and examined the differences in late mortality between these two age groups [71]. The overall standardized mortality ratio (SMR) for the entire cohort was 4.6 (95 % confidence interval (CI) 4.4–4.8). The highest SMRs were for cancer-related deaths including primary and secondary malignancies (SMR = 12.8), followed by infectious diseases (SMR = 4.8), all cardiovascular causes (SMR = 1.9) and cardiac ischemia (SMR 1.9), and respiratory diseases (SMR = 1.7). The majority of the SMRs were higher in the childhood cancer survivors than in the survivors of adult cancer, with overall SMRs of 7.6 and 4.2, respectively. The highest cumulative nonmalignant mortality was due to cardiovascular disease with an ongoing rise throughout the period of follow-up. A second population-based study from the British Columbia Cancer Registry included 1,248 5-year survivors who had been diagnosed with cancer between the ages of 20 and 24 and were assessed for the risk of late mortality and second malignant neoplasms [158]. Compared to the general population, the overall mortality rate was almost six times higher (SMR = 5.9, 95 % CI 4.9–6.9). The most common cause of mortality was due to the primary cancer diagnosis, but the SMR for death from a second malignancy was 5.2 (95 % CI 3.3–7.8) and for noncancer-related deaths was 1.7 (95 % CI 1.1–2.4). These data all highlight the importance of long-term follow-up of AYA survivors with particular attention focused on modifiable risk factors, particularly those related to cardiovascular disease and surveillance for SMNs.
28.2.2 Subsequent Malignant Neoplasms
Subsequent malignant neoplasms (SMN) are a significant cause of morbidity and premature mortality in survivors. Etiological factors for the development of subsequent malignant neoplasms include elements of treatment for the first primary neoplasm – particularly radiotherapy and specific chemotherapies as well as genetic predisposition, hormonal factors, immunosuppression, and the potential interactions between these risk factors. Among survivors of cancer diagnosed in adolescence and young adulthood, there is relatively little opportunity during the initial years of follow-up for environmental factors (e.g., smoking, alcohol, and diet) to be important etiologically, when compared with survivors of primary neoplasms diagnosed in middle age or older adulthood. Both solid cancers and leukemias can present as an SMN in this population.
28.2.2.1 Solid Subsequent Malignant Neoplasms
Among a cohort of over 14,000 survivors of childhood, adolescent, and young adult cancer treated prior to age 21 years, 7.9 % developed an SMN by 30 years after their primary cancer [41]. When compared to the age-matched general population, the risk (measured by standardized incidence ratio [SIR]) was particularly elevated for subsequent cancers of bone, head and neck, thyroid, central nervous system, and soft tissue. The most common subsequent malignancies were breast and thyroid cancers. Exposure to radiation therapy resulted in an almost threefold increase in risk for subsequent cancers.
Even when accounting for radiation, survivors of Hodgkin lymphoma are at particularly elevated risk for development of a solid SMN. Among female survivors of Hodgkin lymphoma treated prior to age 21 years with therapy that includes radiation to the breast, approximately one-third will develop a subsequent breast cancer by age 50 years, a rate comparable to that observed in women with BRCA1 mutations [95]. The risk of breast cancer is also in excess of that expected following Hodgkin lymphoma diagnosed in young adulthood (ages 20–29 years), but the SIRs are lower than for those treated in childhood and adolescence. Unfortunately, lower SIRs are not necessarily accompanied by lower absolute excess risk, as several investigators have reported absolute excess risks of comparable magnitude for patients treated between the ages of 20 and 29 years and below 20 years of age [62]. Breast cancer risk in women who have received chest radiation has been shown to decrease with increasing numbers of alkylating agent cycles or increased radiation dose to the ovaries, suggesting that that hormonal stimulation is important for the development of radiation-induced breast cancer [149, 151]. This mechanism might explain why breast cancer risk declines with age at irradiation for Hodgkin lymphoma; women irradiated at age 30 years or older experience much lower excess risk than those irradiated before this age [62]. Progressive reductions in the extent of the radiation field targeted for Hodgkin lymphoma therapy, and the adoption of new radiotherapy techniques, might reduce the long-term risk for subsequent breast cancers, although further follow-up of survivors treated on contemporary regimens will be needed to accurately quantify reductions in risk [31].
Radiation therapy has also been associated with cancers of the lung, thyroid, stomach, bone, soft tissue, skin, and possibly colon and pancreas in survivors of Hodgkin lymphoma [65]. Doses of radiation to the lungs are substantial as a result of several radiotherapy field configurations used to treat Hodgkin lymphoma. An international collaborative case-control study of the role of radiotherapy, chemotherapy, and smoking in the development of lung cancer after Hodgkin lymphoma reported on 227 patients who developed second primary lung cancer and 455 matched controls who did not [43, 148]. There was a significant increase in the excess risk of lung cancer associated with a greater number of cycles of alkylating agents and increasing radiation dose. Statistically significant elevated risks of lung cancer were apparent within 1–4 years of treatment with alkylating agents, whereas the excess risks after radiotherapy began 5 years after treatment and persisted for more than 20 years. Tobacco use increased lung cancer risk more than 20-fold; risks from smoking appeared to multiply risks from treatment [148].
Recent research has investigated whether there is a genetic predisposition to subsequent malignancies in survivors of Hodgkin lymphoma. One study identified variants in the PRDM1 gene located on chromosome 6q21 as being associated with an increased risk for SMN development after radiation [9]. PRDM1 is a radiation-responsive tumor suppressor gene. Another research is investigating whether polymorphisms in genes associated with drug metabolism or DNA repair and injury response interact with cancer therapies to increase the risk for SMN.
As noted above, the risk for SMN is elevated in survivors of all cancers treated during adolescence and young adulthood but particularly those that have been treated with radiation therapy. Young women treated for a primary breast cancer are one group vulnerable to the development of SMN. Before the implementation of breast-conserving surgery and localized radiotherapy to treat node-negative breast cancer, the principal method of local control was radical mastectomy and extensive radiotherapy to the chest wall and lymph nodes [65]. Consequently, such women have experienced an excess risk of cancers of the contralateral breast and lung and possibly of the esophagus, bone, connective tissue, and thyroid gland [11, 28, 53, 66, 102]. The radiation dose to the contralateral breast can amount to several Gy, and a review [65] has inferred that women irradiated in young adulthood are probably at increased risk of contralateral breast cancer, based mainly on one study [11]. However, another review suggests no convincing evidence of such an effect [30]. Women given radiotherapy who survived at least 10 years appear to have about double the risk of lung cancer experienced by nonirradiated women [66, 102, 147]. This risk is likely to be less following modern radiotherapy techniques. Smoking and radiation have been implicated as risk factors for secondary lung cancer in breast cancer survivors [33, 40].
28.2.2.2 Development of Subsequent Leukemia
Subsequent leukemias (usually acute myeloid leukemia [AML] and myelodysplastic syndrome [MDS]) after cancer therapy have been associated with specific chemotherapy exposures, particularly alkylating agents and topoisomerase II inhibitors (epipodophyllotoxins and anthracyclines). The mean interval between primary cancer and development of secondary AML after alkylating agent exposure is 5–7 years, and AML is often preceded by MDS. Monosomy or partial deletions of chromosomes 5 and 7 are the most common genetic aberrations [59]. In contrast, topoisomerase II inhibitors tend to lead to secondary AML in the first 2–3 years after the primary cancer. These leukemias are usually acute in onset with no preceding MDS and are most frequently associated with rearrangements of the MLL gene on chromosome 11q23 [59]. For example, an analysis of 754 adults treated on Stanford Hodgkin lymphoma protocols over a 30-year period revealed 24 patients (3.2 %) who developed a treatment-related secondary leukemia (particularly AML) or MDS [73], at a median of 4.6 years from their primary cancer. Alkylating agent exposure was demonstrated to be the most important risk factor for secondary leukemia. Males treated for testicular tumors have been shown to be at increased risk for secondary leukemias as a consequence of etoposide exposure [130]. Whereas the risk for solid SMN after a primary cancer does not appear to plateau, the risk for secondary leukemias tends to diminish after the risk period in the first decade after cancer therapy.
The Children’s Oncology Group (COG) has published surveillance guidelines for the detection of SMN in survivors of cancer treated during childhood, adolescence, and young adulthood (available at survivorshipguidelines.org). These include specific recommendations for the types, frequency, and age at initiation for surveillance for breast cancer, colorectal cancer, and skin cancer in survivors deemed to be at high risk (Table 28.2). At the present time, there is no recommendation for routine blood tests to screen for secondary leukemias. Guidelines are reviewed every 5 years, facilitating the addition of new guidelines (such as for lung cancer surveillance) if sufficient evidence exists.
Table 28.2
Surveillance in survivors at high risk for SMN (COG)
Type of SMN | Exposure risk | Screening recommendation |
---|---|---|
Breast | ≥20 Gy radiation therapy to the chest | Annual mammography starting 8 years after radiation or age 25 years, whichever is last |
Colorectal | ≥30 Gy radiation therapy to the abdomen, pelvis, or spine | Colonoscopy every 5 years starting at age 35 year |
Skin | Any radiation therapy | Annual dermatologic examination of irradiated areas |
28.2.3 Cardiovascular Complications
The impact of treatment exposures on cardiovascular (CV) function or disease is twofold, the first being related to exposure to anthracyclines which can classically lead to cardiomyopathy. However, both chemotherapy and radiation can lead to alteration in metabolic functions which contribute to the development of insulin resistance with subsequent development of “metabolic syndrome” and the associated clinical features of hypertension, hyperlipidemia, and hyperinsulinemia. In the general population, these conditions are all known risk factors for the development of cardiovascular disease.
The anthracyclines doxorubicin and daunomycin are well-known causes of cardiomyopathy [48, 129]. Anthracyclines have a wide range of clinical activity, and about 40–50 % of adolescent and young adult cancer survivors were treated with anthracyclines, making them one of the more common treatment exposures. The incidence of cardiomyopathy is dose dependent and may exceed 30 % among patients who received cumulative doses of anthracyclines in excess of 600 mg/m2. With a total dose of 500–600 mg/m2, the incidence is 11 %, falling to less than 1 % for cumulative doses less than 500 mg/m2 [13]. This has formed the basis for considering 500 mg/m2 as the threshold cumulative dose for cardiotoxicity. However, a lower cumulative dose of anthracyclines may place individuals at increased risk for cardiac compromise [75]. The same investigators evaluated the cumulative incidence of anthracycline-induced clinical heart failure in a cohort of 607 patients who had been treated with a mean cumulative anthracycline dose of 301 mg/m2 and were followed for a median of 6.3 years. A cumulative dose of anthracyclines greater than 300 mg/m2 was associated with an increased risk of clinical heart failure (relative risk 11.8) compared with a cumulative dose lower than 300 mg/m2. The estimated risk of clinical heart failure increased with time and approached 5 % after 15 years.
Several investigators have described subclinical anthracycline-induced myocardial damage. Steinherz et al. [136] found 23 % of 201 patients to have echocardiographic abnormalities, a median of 7 years after therapy. The median cumulative dose of doxorubicin received by these patients was 450 mg/m2 (range 200–1,275 mg/m2). Lipshultz and colleagues evaluated cancer survivors who had received a median doxorubicin dose of 334 mg/m2 (range 12–550 mg/m2). They concluded that doxorubicin causes progressive elevation of afterload or depression of left ventricular contractility in about 75 % of the patients. However, the clinical relevance of subclinical myocardial injury is not clearly established, in part due to widely varying methods used to define and assess such injury. These studies and others emphasize that cardiomyopathy can occur many years after completion of therapy (15–20 years) and that the onset may be spontaneous or coincide with exertion or pregnancy. During the third trimester, the cardiac volume increases, increasing the cardiac workload, leading to overt symptoms in women with left ventricular dysfunction [60, 108]. Risk factors known to be associated with anthracycline-related cardiac toxicity include mediastinal radiation (especially doses ≥30 Gy); uncontrolled hypertension; exposure to other chemotherapeutic agents, especially cyclophosphamide; younger age; and female gender [79]. In general higher cumulative doses (≥550 mg/m2 in patients 18 years or older at time of treatment or ≥300 mg/m2 in patients younger than 18 years of age at the time of treatment) are associated with a higher risk of developing clinically significant cardiomyopathy.
Treatment of breast cancer with trastuzumab can also lead to cardiac compromise in up to one-third of patients. In the vast majority of patients, this results in a reversible decrease in LVEF, although some patients may have ongoing heart failure. Patients who have an elevation of troponin levels following trastuzumab may be at higher risk for lack of LVEF recovery [20]. Exposure to higher cumulative doses of anthracyclines (>240 mg/m2 doxorubicin or >500 mg/m2 epirubicin) was found to increase the risk of trastuzumab-induced cardiotoxicity by over threefold [37]. There are no specific recommendations related to the need for any ongoing monitoring in patients in whom LVEF has not been affected or in those who had a reversible decline in LVEF that subsequently normalized. End of therapy assessment of LVEF status is advisable with consideration for additional follow-up if there has been a decline in LVEF from baseline.
Radiation-induced cardiac toxicity can present in a variety of different clinical conditions including congestive heart failure, cardiomyopathy, pericarditis, valvular disease, myocardial infarction, arrhythmia, or atherosclerotic heart disease. Cardiac toxicity associated with radiation alone most commonly involves pericardial effusions or constrictive pericarditis, sometimes in association with pancarditis [1]. Although 40 Gy of total-heart radiation dose appears to be the usual threshold, the risks are also higher in patients who have received anthracyclines with radiation doses ≥30 Gy. However, the risks of pericarditis, congestive heart failure, myocardial infarction, and valvular abnormalities have been reported to be increased by two- to sixfold after as little as 15 Gy compared to nonirradiated survivors [97]. Symptomatic pericarditis, which usually develops 10–30 months after radiation, is found in 2–10 % of patients [92]. Subclinical pericardial and myocardial damage as well as valvular thickening may also be common in this population [110], and there can be a significant latency in the development of symptomatic pericarditis which may first appear as late as 45 years after therapy [51, 127].
Coronary artery disease has been reported following radiation to the mediastinum, although the mortality rate was not significantly higher in patients with Hodgkin lymphoma who had received mediastinal radiation than in the general population [12]. A Dutch study of Hodgkin lymphoma survivors reported a cumulative risk for ischemic heart disease of 21 % at 20 years after radiation [116]. In another study that followed 415 Hodgkin lymphoma survivors, 10 % developed coronary heart disease [64].
Prevention of cardiotoxicity is a primary focus of investigation. Certain analogs of doxorubicin and daunomycin, and liposomal anthracyclines, which appear to have decreased cardiotoxicity, with equivalent antitumor activity, are being explored. The anthracyclines chelate iron, and the anthracycline-iron complex catalyzes the formation of hydroxyl radicals. Agents that are able to remove iron from the anthracyclines, such as dexrazoxane, have been investigated as cardioprotectants. Clinical trials of dexrazoxane have been conducted with encouraging evidence of short-term cardioprotection [16, 155], although the long-term avoidance of cardiotoxicity with the use of this agent needs to be determined. In a prospective, randomized study of pediatric ALL patients, Lipshultz et al. have demonstrated that patients treated with doxorubicin at 300 mg/m2 alone were more likely than those treated with dexrazoxane and doxorubicin to have cardiac injury as reflected by elevated troponin T levels (50 % vs. 21 %, p < 0.001) and extremely elevated troponin T levels (32 % vs. 10 %, p < 0.001), without compromising the antileukemic efficacy of doxorubicin [81]. However, longer follow-up is necessary to determine the influence of dexrazoxane on echocardiographic findings, hence, the clinical significance of these findings. Lower doses of anthracyclines and reduced port sizes of radiation therapy may also help in decreasing the incidence of myocarditis. Management of survivors with asymptomatic deterioration of left ventricular function is controversial. Angiotensin-converting enzyme (ACE) inhibitors have been known to improve morbidity and mortality in patients with cardiomyopathy. There appear to be theoretical risks with such therapy in adolescence, since the ACE inhibitors, while lowering the afterload in the short term, may also limit the cardiac growth potential by inhibiting cardiac growth factors. Thus, the role of ACE inhibitors and beta-blockers in asymptomatic survivors with cardiac dysfunction remains in question [80, 132].
Patients who received anthracycline chemotherapy need ongoing monitoring for late-onset cardiomyopathy, with frequency of evaluation based on total cumulative dose and age at the time of initial therapy. In addition to monitoring for cardiomyopathy, survivors who received radiation potentially impacting the heart (i.e., chest, spine, upper abdomen, or total body irradiation, TBI) also need monitoring for early-onset atherosclerotic heart disease, valvular disease, and pericardial complications. Specific recommendations for monitoring, based on age and therapeutic exposure, are delineated in the COG Long-term Follow-Up Guidelines (described below).
The risk of CV disease can also be related to effects from cancer therapy that impact noncardiac organs/tissues and result in the development of CV risk factors including obesity, dyslipidemia, hypertension, and insulin resistance, all of which are known to be potent risk factors for premature CV disease in adults. While there have not been studies specifically performed in the AYA population, a large study of childhood cancer survivors ≥5 years after diagnosis who were a mean age of 14.5 years when they were carefully evaluated for CV risk and insulin resistance found that compared to siblings, they had greater adiposity (abdominal waist circumference 73.1 vs. 71.1 cm p = 0.02), percent fat (28.1 % vs. 25.9 %, p = 0.007), and lower lean body mass (38.4 vs. 39.9 kg, p = 0.01) [135]. Even after adjusting for adiposity, survivors also were found to have higher total cholesterol (154.7 vs. 148.3 mg/kg, p = 0.004, LDL cholesterol (89.4 vs. 83.7 mg/kg, p = 0.002), triglycerides (91.8 vs. 84 mg/kg, p = 0.03) and were more insulin resistant as measured by euglycemic insulin clamp study (Mlbm 12.1 vs. 13.4 mg/kg/min, p = 0.002) than controls. These findings are significant as CV risk factors track from childhood into adulthood and when already present at such a young age are very likely to contribute to their risk of early CV disease and potentially mortality. A second analysis from the same cohort identified treatment exposures including platinum agents, cranial radiotherapy, and steroids as being associated with insulin resistance and CV risk factors [7]. AYA survivors should have baseline fasting lipid profile and blood glucose obtained at their entry into survivorship. Blood pressure should be monitored on a yearly basis. Counseling regarding the importance of exercise, a nutritionally balanced diet, and maintaining a healthy body weight should be encouraged at each follow-up visit.
28.2.4 Pulmonary Function
Pulmonary fibrosis and pneumonitis can result from pulmonary radiation. Thus, these problems are seen most often in patients with thoracic malignancies, notably Hodgkin lymphoma. Radiation-related pulmonary injuries in AYAs are likely to be mediated by cytokine production, which stimulates septal fibroblasts, increasing collagen production, and resulting in pulmonary fibrosis [72, 122]. Asymptomatic radiographic findings or restrictive changes on pulmonary function testing have been reported in more than 30 % of patients treated with thoracic radiation [61, 89, 103]. Of 25 Hodgkin lymphoma survivors treated with standard mantle radiation before age 35 years, 60 % had an abnormal chest radiograph at a mean follow-up of 9 years [94]. Of the 19 who had pulmonary function testing, 89 % had an abnormality, with 72 % having a reduced diffusion capacity. None of the patients were symptomatic. These changes have been detected months to years after radiation therapy, most often in patients who suffered radiation pneumonitis during or shortly after therapy [154]. Clinically apparent pneumonitis with cough, fever, or dyspnea occurs in only 5–15 % of patients and is generally limited to those who received more than 30 Gy in standard fractions to more than 50 % of the lung [154]. Craniospinal radiation for patients with malignant brain tumors and scatter from abdominal ports contribute to the development of late restrictive lung disease [67]. Obstructive changes have also been reported after conventional radiation therapy. Following hematopoietic stem cell transplantation, both restrictive and obstructive lung diseases including bronchiolitis obliterans are well described [49, 134]. Impaired carbon monoxide diffusing capacity (DLCO) after radiation is predictive of persistent pulmonary morbidity [22]. Refinements in radiation techniques and reductions in doses have decreased the risks for radiation-induced late pulmonary toxicity [54, 152, 157].
In addition to radiation therapy, chemotherapeutic agents are responsible for pulmonary disease in long-term survivors. Bleomycin toxicity is the prototype for chemotherapy-related lung injury, presenting as interstitial pneumonitis and pulmonary fibrosis [107, 133]. The chronic lung toxicity usually follows persistence or progression of abnormalities developing within 3 months of therapy. Like the acute toxicity, it is dose dependent above a threshold cumulative dose of 400 units/m2 and is exacerbated by concurrent or previous radiation therapy [124]. Above 400 units/m2 in the absence of other risk factors, 10 % of patients develop fibrosis [124]. At lower doses, fibrosis occurs sporadically in less than 5 % of patients, with a 1–2 % mortality rate. In some reports, bleomycin toxicity was anticipated on the basis of DLCO abnormalities.
Alkylating agents can also cause chronic lung injury. As with bleomycin, carmustine and lomustine pulmonary toxicity is dose related. Cumulative carmustine doses greater than 600 mg/m2 result in a 50 % incidence of symptoms [6]. A marked increase in pulmonary fibrosis appears at doses exceeding 1,500 mg/m2. Pulmonary fibrosis has also been observed in 16–40 % of transplant recipients treated with cytotoxic conditioning agents including carmustine at doses of 500–600 mg/m2; the incidence of fibrosis declines considerably when doses are limited to less than 300–450 mg/m2 [123, 156]. Case reports and small series suggest that cyclophosphamide can cause delayed-onset pulmonary fibrosis with severe restrictive lung disease in association with a marked reduction in the anteroposterior diameter of the chest [8, 24]. Melphalan and busulfan are also known to cause pulmonary fibrosis in a dose-related manner. Busulfan toxicity is most predictable in transplantation doses exceeding 500 mg and may be associated with a progressive, potentially fatal restrictive lung disease. Lung injury associated with busulfan is characterized by diffuse interstitial fibrosis and bronchopulmonary dysplasia.
Additional factors contributing to chronic pulmonary toxicity include superimposed infection, underlying pneumonopathy (e.g., asthma), cigarette or respirator toxicity, chronic graft versus host disease, and the effects of chronic pulmonary involvement by tumor or reaction to tumor. Increased oxygen concentrations associated with general anesthesia may exacerbate pulmonary fibrosis, and efforts should be made to minimize exposure to high concentrations of oxygen [46]. There is controversy as to whether the high oxygen pressure associated with scuba diving can exacerbate pulmonary damage [78, 126].
Monitoring for pulmonary dysfunction in cancer survivors includes asking about symptoms such as chronic cough or dyspnea on yearly follow-up. All patients must understand the risks of smoking. The best approach to chronic pulmonary toxicity of anticancer therapy is preventive and includes respecting cumulative dosage restrictions of bleomycin and alkylators, limiting radiation dosage and port sizes, and avoiding primary or secondhand smoke. Pulmonary function tests (including DLCO and spirometry) have been recommended as a baseline upon entry into long-term follow-up for patients at risk, in patients with symptoms, or in those who require general anesthesia for any reason.
28.2.5 Endocrine Function
The endocrine system is particularly susceptible to the long-term effects of cancer therapy. In a survey of the patients attending one late effects clinic, 41 % had an endocrinopathy directly attributable to their disease or treatment. This is almost certainly an underestimate as it did not take into account the risk of growth hormone (GH) deficiency which has since been recognized to have important implications in adult life. Within the same group, a further 14 % were reported to have problems related to fertility [137]. The endocrine system is particularly affected by radiotherapy which impacts the normal function of the hypothalamic-pituitary axis, the thyroid, and the gonads. Chemotherapy can have a significant effect upon gonadal function affecting steroid hormone secretion and reproductive potential. The recent introduction of biological agents that modulate the immune system inducing an autoimmune reaction in the tumor has seen an advance in the way cancer may be treated; however, these agents are associated with significant endocrine side effects.
28.2.6 Pituitary Function
Hypopituitarism, deficiency of one of more anterior pituitary hormones [gonadotrophins (GH), follicle-stimulating hormone (FSH) and luteinizing hormone (LH), adrenocorticotrophic hormone (ACTH), and thyroid-stimulating hormone (TSH)], may be present at diagnosis caused by pathology in the sellar or suprasellar region, which destroys normal pituitary tissue or disrupts the pituitary stalk, or by the treatments used, either surgery or irradiation. Deficiencies of the posterior pituitary hormones, antidiuretic hormone (ADH) and oxytocin, may occur in the presence of large suprasellar lesions such as a craniopharyngioma or germinoma, but are not caused by irradiation [26].
Patients at risk of radiation-induced hypopituitarism may have been treated for an intracranial tumor, malignancy of the nasopharynx, and ALL or with radiation as preparation for blood or marrow transplantation [45]. Pituitary dysfunction may develop several years after treatment and can be progressive; GH secretion is the most vulnerable to irradiation, followed by the gonadotrophins, ACTH, and finally TSH [76, 82]. The risk of hypopituitarism increases with time from radiation and as the radiation dose increases [145, 146]. Patients treated for ALL, the most common childhood malignancy, have been found to have abnormalities of GH secretion up to 25 years after they received prophylactic cranial irradiation at doses of 18–24 Gy [15]. Patients exposed to higher doses, such as those used to treat nasopharyngeal tumors or malignant brain tumors, are at greater risk; 50 % will have abnormal GH secretion within 5 years of treatment, and many will go on to develop other abnormalities of anterior pituitary function [76].
Cytotoxic chemotherapy has not been shown to have a clinically significant effect on pituitary function in cohort studies. However, immunotherapies used in adults have caused autoimmune hypophysitis and hypopituitarism. These antibodies, so-called immune checkpoint inhibitors, disrupt the immune system generating an immune reaction against the tumor [112]. One such agent, ipilimumab, is directed against the cytotoxic T-lymphocyte antigen-4 receptor (CTLA4) enhancing immunological antitumor activity by decreasing tumor immune tolerance [25]. Up to 17 % of patients treated with ipilimumab develop hypophysitis. The effect is dose dependent. Management includes suspending treatment and replacing affected endocrine axes. High-dose glucocorticoid treatment has been used in an attempt to reduce the impact of the hypophysitis, but the benefit is questionable.
The majority of patients diagnosed with malignant disease between the ages of 15 and 39 years will have completed growth and development. However, GH is now known to play an important role throughout the adult lifespan but particularly up to the age of 25 years. Studies have shown that GH-deficient adults complain of fatigue, have abnormal body composition (fat mass is increased and lean mass decreased), are osteopenic [27], and exhibit an adverse cardiovascular risk profile, which may contribute to the twofold increased cardiovascular mortality observed in patients with hypopituitarism [144]. GH replacement therapy, administered to adults as a single nightly injection, improves quality of life, increases lean mass, decreases fat mass, increases bone mineral density, and improves the cardiovascular risk profile. Although the improvements in the cardiovascular risk profile would support an improvement in cardiovascular risk, it is not yet known whether GH replacement therapy reduces mortality in adults [145].
GH is important for skeletal health, particularly in the years immediately after achieving final height, when it is vital to optimize peak bone mass, which is achieved in the middle of the third decade. A study in adolescents treated for GH deficiency during childhood has shown that continuing GH replacement beyond achievement of final height doubles the rate of bone mass accrual [128]. Thus, young adults that develop GH deficiency may not reach peak bone mass, which will increase their risk of osteoporosis in the future. In a patient cohort that may have been exposed to other agents that have a negative impact upon bone mass, such as high-dose glucocorticoids, it is important to ensure that peak bone mass is achieved in order to minimize the risk of fracture in later life.
28.2.7 Gonadal Function
The ovaries and testes are both sensitive to the effects of chemotherapy and radiotherapy. The risk of premature ovarian failure increases as the age at treatment increases or when treatment contains radiation below the diaphragm with alkylating agent chemotherapy. Treatment before the age of 13 is less likely to be associated with an increased risk, but treatment between the ages of 13 and 19 was associated with a twofold increase in risk of developing premature ovarian failure [17, 18]. This risk increases further as the age of the women at diagnosis increases. The majority of adolescent women undergoing treatment with combination chemotherapy will retain ovarian function. However, women undergoing bone marrow transplant are at particular risk of ovarian failure. Some series suggest that the frequency of ovarian failure in women pretreated with high-dose alkylating agents may be as high as 100 % [125].
The ovaries are particularly sensitive to radiation. Abdominal irradiation for Hodgkin lymphoma or Wilms’ tumor is associated with a high risk of ovarian failure. TBI used in preparation for blood and marrow transplantation is associated with ovarian failure in 100 % of women at the time of treatment, of whom a small number will experience subsequent recovery of function [45].
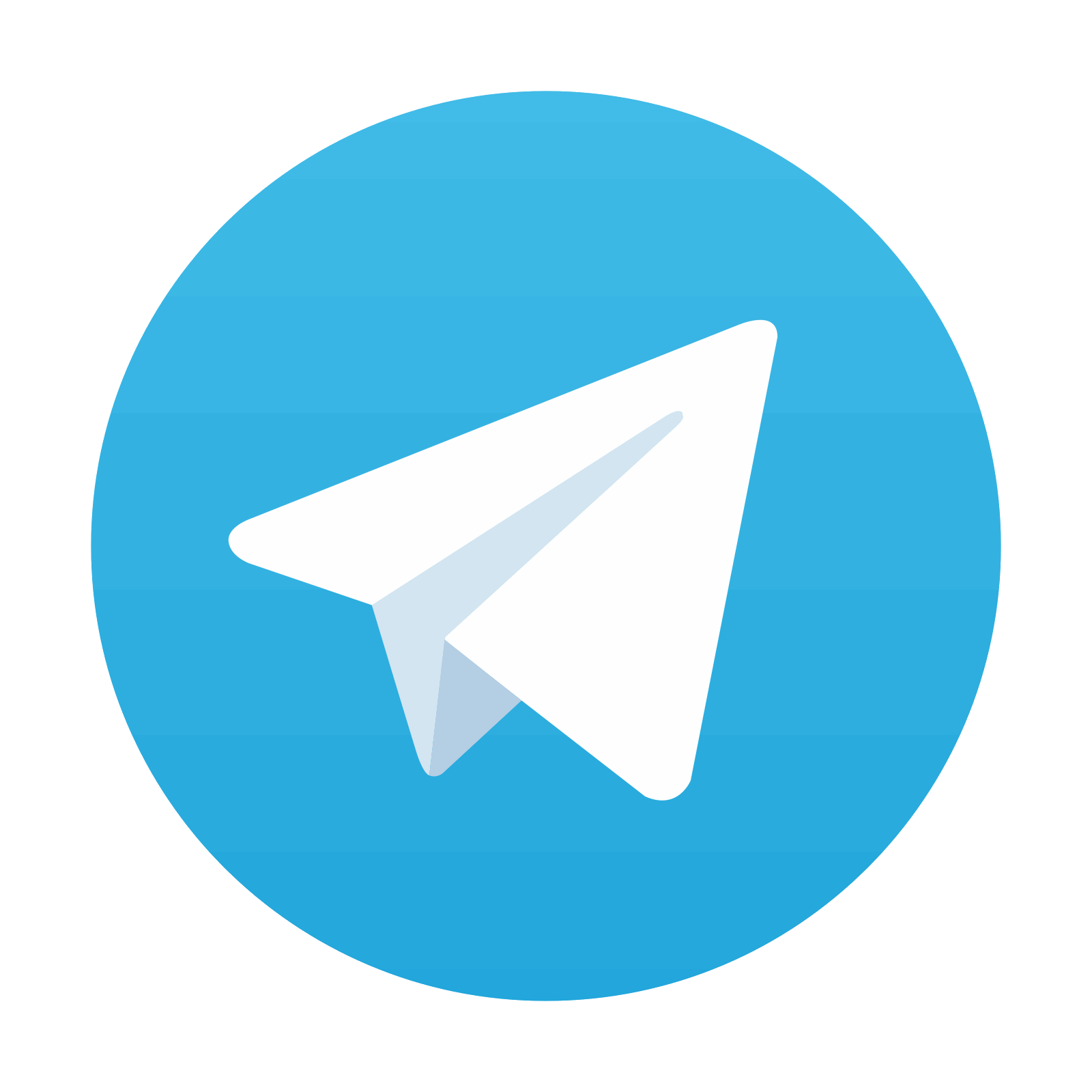
Stay updated, free articles. Join our Telegram channel

Full access? Get Clinical Tree
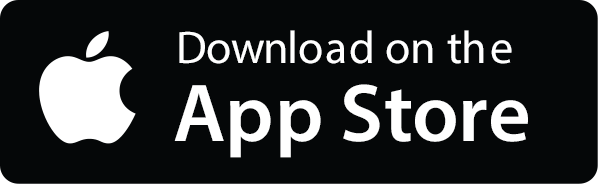
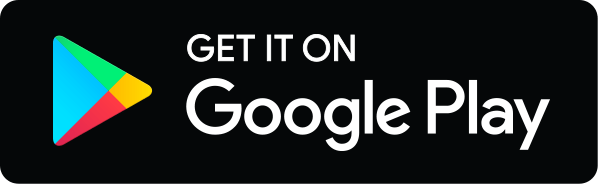