M.A. Hayat (ed.)Stem Cells and Cancer Stem CellsStem Cells and Cancer Stem Cells, Volume 122014Therapeutic Applications in Disease and Injury10.1007/978-94-017-8032-2_15
© Springer Science+Business Media Dordrecht 2014
15. Adipose-Derived Stem Cells as a Novel Tool for Future Regenerative Medicine
(1)
Department of Plastic and Reconstructive Surgery, Juntendo University School of Medicine, 2-1-1 Hongo, Bunkyo-ku, Tokyo 1138421, Japan
(2)
Department of Surgery, Surgical Bio-engineering Laboratory, University of California, Davis, 4625 2nd Avenue, Room 3001, Sacramento, CA 95817, USA
(3)
Department of Plastic and Reconstructive Surgery, Baskent University Faculty of Medicine, Fevzi Cakmak Cad. 5. Sok., No: 48, 06490 Bahcelievler, Ankara, Turkey
(4)
Department of Plastic and Reconstructive Surgery, Nanfang Hospital of Southerm Medical University, Guangzhou Dadao Bei 1838, Baiyun District, Guangzhou, China
Abstract
Stem cell-based therapies for the repair and regeneration of damaged tissues and organs offer a paradigm shift that may provide alternative therapeutic solutions for a number of diseases. The use of either embryonic stem cells or induced pluripotent stem cells in clinical situations may be still limited due to cell behaviors, ethical considerations, and genetic manipulation, even though these cells are theoretically highly beneficial. Adipose-derived stem cells (ASCs) seem to be an ideal population of stem cells for practical regenerative medicine since they are plentiful, of autologous tissue origin and thus non-immunogenic, and are more available due to minimal ethical considerations. Furthermore, recent basic research and preclinical studies have revealed that the use of ASCs in regenerative medicine is not limited to mesodermal components, but extends to both ectodermal and endodermal tissues and organs, even though ASCs are mesodermal in origin. This chapter will describe the biology of ASCs and their proliferation and differentiation capacities, and will summarize the current preclinical and clinical data from a variety of medical fields on the use of ASCs in regenerative medicine.
Introduction
Promising bioengineering technologies such as tissue engineering, which is an interdisciplinary field involving physicians, engineers and scientists, may provide novel tools for reconstructive surgery. A number of life-threatening conditions, such organ failure, tissue loss due to trauma, cancer, or congenital structural anomalies, can be treated by current clinical procedures/surgical strategies that include organ transplantation, autologous tissue transfer, and the use of artificial materials. However, these treatments have potential limitations, including organ shortages, donor site morbidity in harvesting autologous tissues, allergic reactions, and immune rejection. Recent developments in the emerging field of stem cell science, stem cell-associated growth factors, and regenerative medicine may allow the use of stem cells to repair tissue damage and, eventually, to replace organs.
A stem cell is characterized by its ability to self-renew and to differentiate along multiple lineage pathways. Candidate stem cells include embryonic stem cells (ESCs), induced pluripotent stem cells (iPSCs), and adult stem cells. ESCs are capable of extensive self-renewal and expansion, and have the potential to differentiate into any type of somatic tissue. These traits make human ESCs promising for future use in regenerative medicine. The iPSCs are derived from differentiated cells such as skin fibroblasts and appear to have the same potential and properties as ESCs; however, their generation is not dependent upon a source of embryos. As such, although the therapeutic potential of both ESCs and iPSCs is enormous due to their self-renewal and pluripotent features, there are limitations to their practical use such as the regulation of teratoma formation, ethical considerations, and immune responses to ESCs, as well as difficulties with genetic manipulation of iPSCs. By contrast, adult stem cells are, by their nature, immunocompatible, and there are no ethical concerns related to their use.
Multipotent mesenchymal stem cells (MSCs) are non-hematopoietic cells of mesodermal origin that are present in a number of postnatal and adult organs and connective tissues. To date, MSCs with characteristics similar to bone marrow-derived MSCs have been isolated from different tissue sources including trabecular bone, periosteum, synovial membrane, skeletal muscle, skin, pericytes, peripheral blood, deciduous teeth, periodontal ligament and umbilical cord. Although the stem cell populations derived from these sources are variable, common problems include limited amounts of harvested tissues and low numbers of harvested cells. With such a limited supply of stem cells, ex vivo expansion or further manipulation would be required to obtain a sufficient quantity of cells for clinical applications, and the efficacy and safety of such procedures would require verification before they could be used clinically.
Adipose tissue is present in abundance in many stores throughout the body, where it plays a major role in energy balance and displays strong endocrine functions. The multipotent stem cells within adipose tissue, termed adipose-derived stem cells (ASCs) (Zuk et al. 2001), are one of the most promising stem cell populations since human adipose tissue is ubiquitous and easily obtained in large quantities with little donor site morbidity or patient discomfort. Therefore, the use of autologous ASCs both as research tools and for cellular therapeutics is feasible, and ASCs are both safe and efficacious, as shown by preclinical and clinical studies of injury and disease. To date, the biology of ASCs has been described, preclinical studies of the use of ASCs in regenerative medicine have been performed, and the efficacy of ASCs has been determined in several clinical trials. Therefore, this chapter will highlight recent progress in the preclinical and clinical application of ASCs in various medical fields, as well as summarize our understanding of ASC biology, and discuss future directions for the use of ASCs with particular reference to the field of regenerative medicine.
Localization and Isolation of ASCs
Adipose tissue is a highly complex tissue consisting of mature adipocytes, which constitute more than 90% of the tissue volume, and a stromal vascular fraction (SVF), which includes preadipocytes, vascular smooth muscle cells, endothelial cells, fibroblasts, resident monocytes/macrophages, lymphocytes and ASCs. Many of the characteristics of ASCs may differ according to the location of the harvested adipose tissue. The density of stem cells in adipose tissue is also variable and is a function of location, tissue type, and species. In white adipose tissue, ASC yields are greater in subcutaneous deposits compared to visceral fat, with the highest concentration of ASCs in arm adipose tissue deposits. ASCs from inguinal adipose tissue deposits exhibit the greatest plasticity (Prunet-Marcassus et al. 2006). ASCs are also found within brown adipose tissue deposits distributed within white fat deposits, and these ASCs readily undergo skeletal myogenic differentiation.
Stem cell yields are greater from adipose tissue than from other stem cell reservoirs, which is another significant factor in their suitability for use in regenerative medicine. Approximately 1 × 107 adipose stromal/stem cells can be routinely isolated from 300 ml of lipoaspirate with greater than 95% purity (Boquest et al. 2006). The average frequency of ASCs in processed lipoaspirate is 2% of nucleated cells, and the yield of ASCs is approximately 5,000 fibroblast colony-forming units (CFU-F) per gram of adipose tissue, compared with estimates of approximately 100–1,000 CFU-F per milliliter of bone marrow.
Subcutaneous adipose tissue can be easily obtained under local anesthesia. Current methods for isolating ASCs rely on collagenase digestion followed by centrifugal separation to isolate the SVF from primary adipocytes. The ASCs exhibit a fibroblast-like morphology and lack the intracellular lipid droplets observed in adipocytes. Isolated ASCs are typically expanded in monolayer culture on standard tissue culture plastics with a basal medium containing 10% fetal bovine serum.
Cellular Characteristics of ASCs
Freshly-isolated SVF cells are a heterogeneous cell population that includes putative ASCs (CD31−, CD34+/−, CD45−, CD90+, CD105−, and CD146−), vascular smooth muscle cells or pericytes (CD31−, CD34+/−, CD45−, CD90+, CD105−, and CD146+), endothelial progenitor cells (CD31+, CD34+, CD45−, CD90+, CD105−, and CD146+), and hematopoietic cells (CD45+) in uncultured conditions (Zimmerlin et al. 2010). In addition, freshly-isolated SVF cells and early passage ASCs express higher levels of CD117 (c-kit), HLA-DR, and stem cell-associated markers such as CD34, and lower levels of stromal cell markers such as CD13, CD29 (β1 integrin), CD44, CD63, CD73, CD90, CD105 and CD166 compared to ASCs from later passages (Bailey et al. 2010). While the consequences of the decrease in CD34 expression in later passage ASCs are not clear, there is at least one study demonstrating that CD34 expression can be maintained during 20 weeks of culture (Yoshimura et al. 2006). In addition, several studies have shown that CD34+ ASCs have a greater proliferative capacity, while CD34- ASCs have greater plasticity.
As indicated above, ASCs share many cell surface markers with pericytes and bone marrow-MSCs. In addition to those mentioned above, the pericyte markers expressed by ASCs include smooth muscle β-actin, platelet-derived growth factor receptor-β (PDGF-β), and neuro-glial proteoglycan 2 (NG2), while the markers shared by ASCs and MSCs include CD13, CD29, CD44, CD58 and CD166. The exact location of ASCs within adipose tissue remains elusive; however, several studies have suggested that ASCs may exist within the perivascular tissue since they express cell surface antigens that are similar to pericytes.
Culturing of ASCs eventually results in the appearance of a relatively homogenous population of mesodermal or MSCs. However, the different techniques used for harvesting ASCs greatly affect the proportions of various cell types that accompany them. Furthermore, cell culture conditions markedly affect ASC gene expression profiles; particularly important are the medium used and the mechanophysiological environment (e.g., three-dimensional culture, the imposition of mechanical force on the cells, and the degree of oxygenation).
ASCs exhibit elevated telomerase activity. Although the activity is lower than that in cancer cell lines, the level of telomerase activity indicates that ASCs have the capacity for self-renewal and proliferation (Jeon et al. 2011). Finally, Puissant et al. reported that human ASCs lack HLA-DR expression and have immunosuppressive properties (Puissant et al. 2005). Based on such findings, preliminary clinical data demonstrated that severe steroid-refractory acute graft-versus-host disease (GVHD) could be treated with human ASCs from HLA-mismatched donors.
Proliferation Capacity of ASCs
The proliferative capacity of ASCs is greater than that of bone marrow-derived MSCs. The doubling time of ASCs during the logarithmic phase of growth ranges from 40 to 120 h, and depends on donor age, tissue type (white or brown adipose tissue) and location (subcutaneous or visceral), harvesting technique, culture conditions, plating density, and media formulations. The younger the donor, the greater the proliferation and cell adhesion of the ASCs. With repeated passaging, the cells gradually lose their proliferative capacity. Cell senescence of ASCs, based on β-galactosidase activity, is similar to that of bone marrow-derived MSCs.
The proliferation of ASCs can be stimulated by application of individual growth factors such as fibroblast growth factor (FGF-2), epidermal growth factor (EGF), insulin-like growth factor 1 (IGF-1), or tumour necrosis factor α (TNF-α). Particularly, FGF-2 is an effective growth-stimulating factor and is required for the long-term propagation and self-renewal of ASCs via the extracellular signal-related kinase 1/2 (ERK1/2) signalling pathway (Zaragosi et al. 2006). The proliferation of ASCs can be also stimulated by PDGF via activation of Jun amino-terminal kinase (JNK) and by Oncostatin M via activation of the microtubule-associated protein kinase/extracellular signal-regulated kinase and the JAK3/STAT1 pathways. Proliferation of ASCs is also enhanced by multiple growth factors, which can include any of the single growth factors mentioned above supplemented by thrombin-activated platelet-rich plasma, human platelet lysate and human thrombin (Kakudo et al. 2008).
ASCs are generally considered to be stable in long-term culture. ASCs that underwent more than 100 population doublings retained a normal diploid karyotype (Rodriguez et al. 2004). One report suggested that human ASCs undergo malignant transformation when passaged for more than 4 months, but recent reports show that spontaneous transformation of MSCs may be due to cross-contamination with malignant cell lines such as fibrosarcoma and osteosarcoma lines. Since the issue of spontaneous ASC transformation is still controversial, further experiments and discussion are required, and careful manipulation of ASCs and long-term observation of patients after clinical use of ASCs are essential.
Differentiation Potential of ASCs to Various Lineages
Numerous reports have demonstrated that ASCs possess the potential to differentiate into a variety of cell lineages both in vitro and in vivo. Although ASCs are obviously of mesodermal origin, it is now clear that they can differentiate not only into mesodermal lineages, but also into ectodermal and endodermal lineages.
ASCs can differentiate into many mesodermal lineages, including adipogenic, osteogenic, chondrogenic, myogenic, cardiomyogenic, angiogenic, tenogenic and periodontogenic lineages, and tissue regeneration studies with suitable scaffolds and growth factors in an appropriate external environment have been conducted. Survival of fat tissue grafts is limited and, thus, is associated with inconsistent clinical outcomes. The transplanted fat graft loses volume over time due to tissue resorption that can result in the loss of 20–90% of the original graft volume. Graft loss is mainly attributed to a lack of vascularization within the new tissue, leading to cell necrosis. Recently, the use of grafts containing ASCs and aspirated fat, called cell-assisted lipotransfer (CAL), was reported (Matsumoto et al. 2006). In theory, this type of approach could accomplish several desired effects, including (1) direct differentiation of ASCs into adipocytes as a reservoir for adipose tissue turnover; (2) direct differentiation of ASCs into endothelial cells and, subsequently, an increase in blood supply to the grafted fat tissue, thereby decreasing the rate of graft resorption; (3) release of angiogenic growth factors by ASCs and induction of angiogenesis; (4) protection of the graft from ischemic reperfusion injury by ASCs; and (5) acceleration of wound healing at the recipient site.
There have been a number of publications on ASC-based bone engineering and regeneration since the first report showing ASC-driven osteogenesis in vivo (Cowan et al. 2004). Different reports have examined various features of bone regeneration, including different scaffolds and methods for osteogenic induction. Currently, several scaffolds are being used in preclinical bone engineering studies, including hydroxyapatite, beta-tricalcium phosphate (β-TCP), polylactide (PLA), polyglycolide (PGA), poly (lactic-co-glycolic) acid (PLGA), anorganic bovine bone (ABB), and composite scaffolds. Exogenous administration of bone morphogenetic protein-2 (BMP-2) is also promising for bone tissue engineering and regeneration.
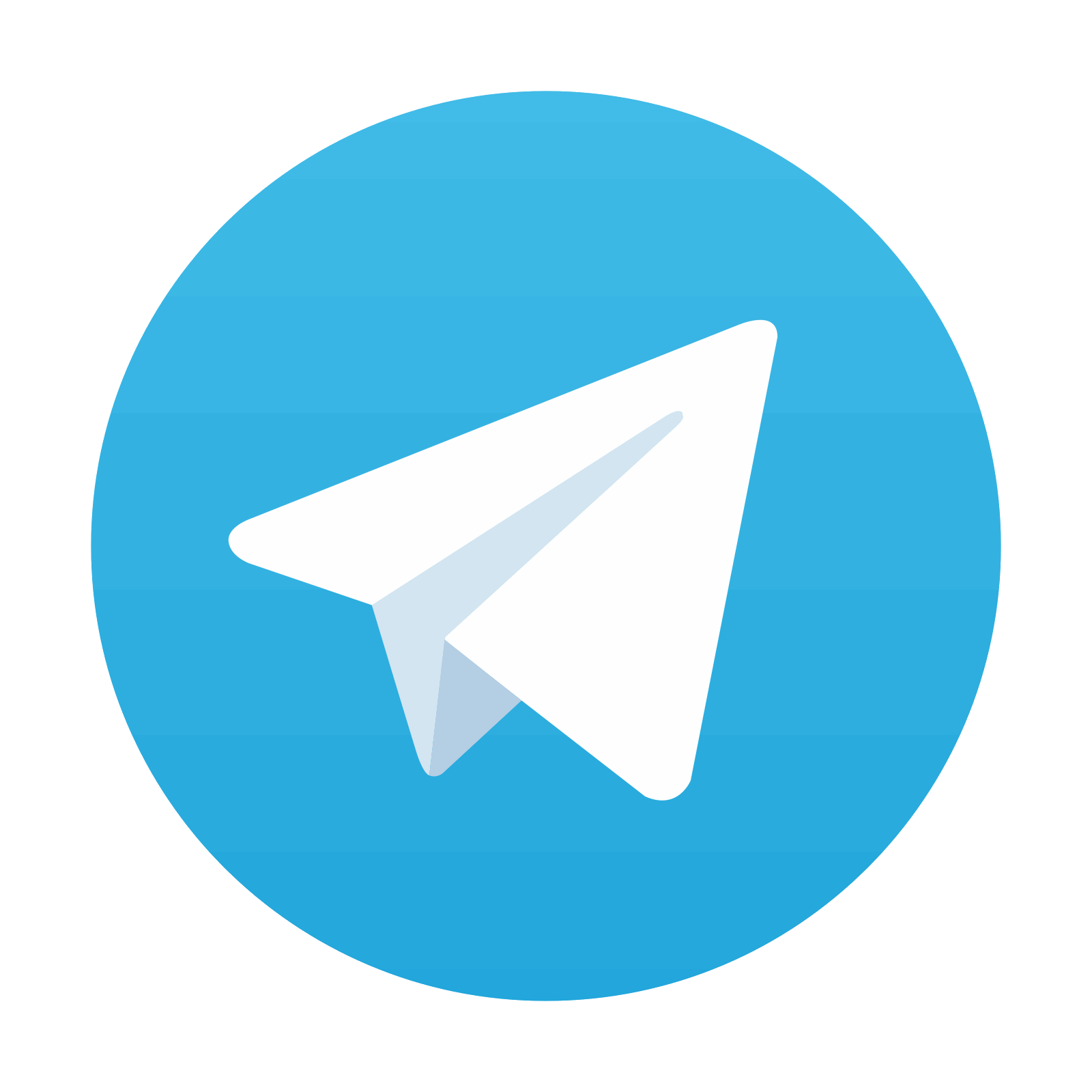
Stay updated, free articles. Join our Telegram channel

Full access? Get Clinical Tree
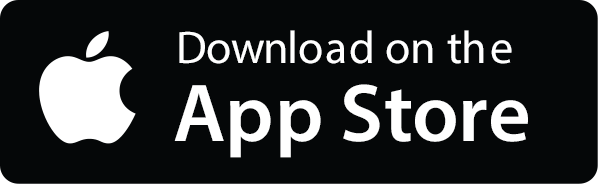
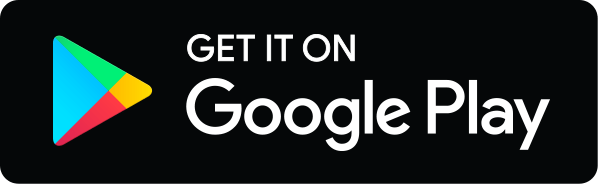