Chapter Outline
Acquired and congenital bone marrow failure syndromes are characterized by a reduction in the effective production of mature erythrocytes, granulocytes, and platelets by the bone marrow. Bone marrow failure leads to various peripheral blood cytopenias. In some conditions, only one or two cell lines may be affected. In others such as aplastic anemia the result is pancytopenia. In this chapter, we deal only with the acquired bone marrow failure syndromes, though we use the term rather loosely because the acquired marrow failure syndromes may have a genetic basis. The known inherited/congenital bone marrow failure syndromes are described in detail in Chapter 7 . The acquired marrow failure syndromes that affect only platelets or granulocytes are described in Chapters 22 and 34 respectively. The acquired single lineage deficiency syndromes that involve red cell production (pure red cell aplasia) are also described in this chapter, except that the 5Q-syndrome is discussed in Chapter 7 and in more detail Chapter 51 .
Aplastic Anemia
Decreased production of mature blood cells may result from a reduction in the number or function of their progenitors . Aplastic anemia is a descriptive term referring to a clinical state in which peripheral blood pancytopenia results from reduced or absent production of blood cells in the bone marrow. Aplastic anemia may arise in the setting of inherited/congenital syndromes associated with a predisposition to marrow failure (discussed in Chapter 7 ), may develop secondary to marrow-toxic stressors in an otherwise seemingly normal host, or may lack any apparent underlying cause. Classifications of the aplastic anemias are presented in Box 6-1 . A careful medical history, physical examination, and laboratory evaluation are critical to discern inherited versus acquired causes of aplastic anemia. The distinction between inherited and acquired causes of aplastic anemia carries profound implications for medical management and treatment, so a careful search for possible underlying inherited syndromes should be undertaken prior to initiation of therapy.
Acquired
Secondary
Radiation
Drugs and chemicals
Direct toxicity: chemotherapy; benzene
Idiosyncratic: chloramphenicol; antiinflammatory drugs; antiepileptics; carbonic anhydrase inhibitors
Viruses
Epstein-Barr virus
Hepatitis (non-A, B, C, E, or G)
Human immunodeficiency virus
Immune diseases
Eosinophilic fasciitis
Hypoimmunoglobulinemia
Systemic lupus erythematosis (uncommon)
Thymoma
Graft-versus-host disease in immunodeficiency
Pregnancy
Paroxysmal nocturnal hemoglobinuria
Myelodysplasia
Idiopathic
Inherited
Fanconi anemia
Dyskeratosis congenita
Shwachman-Diamond syndrome
Amegakaryocytic thrombocytopenia
Diamond-Blackfan anemia
Reticular dysgenesis
GATA-2 syndromes
Familial aplastic anemias
Nonhematologic syndromes (e.g., Down, Dubowitz, and Seckel syndromes)
Camitta and co-workers classified the severity of aplastic anemia in an effort to make possible the comparison of diverse groups of patients and different therapeutic approaches. Diagnosis of severe aplastic anemia requires that the patient have at least two of the following anomalies: a granulocyte count below 500/µL, a platelet count below 20,000/µL, and an absolute reticulocyte count less than or equal to 40 × 10 9 /L . In addition the bone marrow biopsy must contain less than 25% of the normal cellularity or less than 30% hematopoietic elements ( Fig. 6-1 ). Very severe aplastic anemia is further defined by a granulocyte count of less than 200/µL. Mild or moderate aplastic anemia, sometimes called hypoplastic anemia, is distinguished from the severe form by the presence of mild or moderate cytopenias and more variable, but still deficient, bone marrow cellularity. These distinctions are more than semantic; they are critical for the prediction of outcome and the choice of therapy.
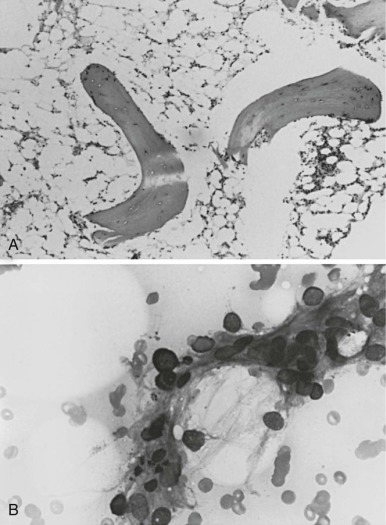
Epidemiology
Epidemiologic studies performed in Europe estimate that the annual incidence of aplastic anemia is 2 per million per year. By comparison, the incidence of acute leukemia is about 50 per million per year. Higher figures for the incidence of aplastic anemia have been obtained from smaller studies in the United States and earlier surveys in Europe, but these figures may have been inflated by the inclusion of cases of myelodysplasia, a much more common syndrome. Aplastic anemia is more common in Asia (4 to 7 per million per year) than in the West. Chloramphenicol, a known cause of aplastic anemia, has been widely used in much of Asia because of its efficacy and low cost, but reductions in its use have not been accompanied by reductions in aplastic anemia incidence in Japan or elsewhere, and no association was observed in the case control studies in Thailand. A recent case control study in Thailand spanning 1989-2002 described an association between aplastic anemia and exposure to benzene or pesticides. Although an increased risk of aplastic anemia was also associated with animal exposures and ingestion of nonbottled or nondistilled water, no significant associations with known infections or hepatitis were observed.
The peak age of presentation of aplastic anemia is at 15 to 25 years or over 60 years. The male-to-female ratio in acquired aplastic anemia is approximately 1 : 1.
Causal Factors
When no causative factors are ascertained, the cases are classified as idiopathic. A search for possible causative agents is warranted because some patients may improve following the removal of the offending agent. However, most cases of aplastic anemia remain idiopathic. The distinction between acquired versus inherited causes of aplastic anemia is crucial to guide clinical management and treatment (see Chapter 8 ).
Drugs
The incidence of drug- and chemical-related aplastic anemia varies over time and from place to place. Many drugs and toxins have been implicated by inferential and circumstantial evidence; the magnitude of the risk is usually unknown ( Box 6-2 ). Presence of an agent on this list suggests caution regarding its use, but no drug on this list should be proscribed if there are strong clinical indications for its use. From a public health perspective, even drugs associated with an increased risk of marrow failure do not cause large numbers of cases of aplastic anemia.
* Agents are listed because they have been cited in the literature; inclusion in this list does not imply acceptance by the author of a causal relationship.
Agents That Regularly Produce Marrow Depression
Antibiotics: daunorubicin, doxorubicin hydrochloride (Adriamycin), chloramphenicol
Antimetabolites: antifolic compounds, nucleotide analogues
Antimitotics: vinblastine, colchicine
Benzene and chemicals containing benzene: carbon tetrachloride, chlorophenols, kerosene, Stoddard solvent
Cytotoxic cancer chemotherapy with alkylating drugs: busulfan, melphalan, cyclophosphamide
Agents Possibly Associated but with a Low Probability Relative to Use
Chloramphenicol
Insecticides: chlordane, chlorophenothane (DDT), γ-benzene hexachloride (lindane), parathion
Anticonvulsants: carbamazepine, hydantoins, phenacemide
Nonsteroidal antiinflammatory agents: indomethacin, ibuprofen, oxyphenylbutazone, phenylbutazone, sulindac
Antihistamines: cimetidine, chlorpheniramine, ranitidine
Antiprotozoal drugs: quinacrine, chloroquine
Sulfonamides: some antibiotics, antidiabetics (chlorpropamide, tolbutamide), antithyroid drugs (methimazole, methylthiouracil, propylthiouracil), carbonic anhydrase inhibitors (acetazolamide, methazolamide)
Penicillamine
Metals: gold, arsenic, bismuth, mercury
Agents More Rarely Associated
Allopurinol (may potentiate marrow suppression by cytotoxic drugs)
Antibiotics: flucytosine, mebendazole, methicillin, sulfonamides, streptomycin, tetracycline, trimethoprim/sulfamethoxazole
Carbimazole
Guanidine
Lithium
Methyldopa
Potassium perchlorate
Quinidine
Sedatives and tranquilizers: chlordiazepoxide, chlorpromazine, meprobamate, methyprylon, piperacetazine, prochlorperazine
Thiocyanate
Note that even confirmed associations do not substantiate causality. Antibiotics felt to be causative may have been administered for the viral infection that led to the aplastic anemia or for symptoms from already established neutropenia in an undiagnosed case of aplastic anemia. Bleeding may be precipitated in undiagnosed thrombocytopenic patients who receive nonsteroidal antiinflammatory drugs. As an example of known errors in associations, among six patients reported to have sniffed glue and become aplastic, five had sickle cell anemia and aplastic crises now known to be due to parvovirus infection.
The incidence of drug-related aplasia in pediatric cases is low, mainly because many of the drugs felt to be related to aplasia are not used in childhood, with the exception of antiepileptic drugs, carbonic anhydrase inhibitors, nonsteroidal antiinflammatory medications, and some antibiotics.
Drug-related aplasia may occur in several ways. Drugs may exert direct cytotoxic or suppressive effects on the bone marrow. Myelosuppressive drugs, such as those used in cancer chemotherapy, lead to predictable and dose-related marrow suppression. Benzene, too, can be demonstrated regularly to suppress the bone marrow in animals in a dose-linked manner, and most individuals exposed to sufficient amounts of benzene would probably suffer some type of marrow damage. In practice, most drug-related aplastic anemia is idiosyncratic and occurs unpredictably in only rare individuals who are prescribed the medication, sometimes weeks to months after its administration is discontinued. This last category of patients may possess a genetic propensity for this phenomenon or be due to a direct effect of the drug on antigen presentation of abnormal peptides (see below).
Chloramphenicol
Chloramphenicol was considered to be the commonest cause of aplastic anemia at the peak of its use, which began in 1949. A genetic predisposition may exist. The mechanism of the idiosyncratic aplasia remains unknown despite extensive investigation.
Chloramphenicol contains a nitrobenzene ring and thus resembles amidopyrine, a drug known to cause agranulocytosis. Chloramphenicol is the prime example of a drug that causes both dose-related marrow suppression through mechanisms that include mitochondrial inhibition, and idiosyncratic aplastic anemia.
The signs of dose-related toxicity appear more rapidly in patients with hepatic or renal disease because the drug must be inactivated by conjugation with glucuronide in the liver and excreted in the urine. High doses and high plasma levels correlate with the characteristic reversible erythroid depression. In vitro, chloramphenicol inhibits the growth of both colony-forming units granulocyte/macrophage (CFU-GMs) and colony-forming units erythroid (CFU-Es) and also may inhibit the hematopoietic microenvironment (HM).
Other Drugs
Nonsteroidal antiinflammatory drugs, which are used more extensively in adults than in children, are associated with aplasia. Nonsteroidal antiinflammatory drugs associated by occasional case reports with aplastic anemia include aspirin, indomethacin, and ibuprofen. Several large studies reported an increased risk of aplastic anemia with phenylbutazone and identified even higher probabilities with some of the other nonsteroidal antiinflammatory drugs. Cimetidine, another commonly used drug, is associated with a 2 per 100,000 user risk of cytopenias. Sulfa-containing compounds, which appear as risk factors in most case-control studies of drugs and aplastic anemia, are used in a wide variety of clinical circumstances. Other drugs implicated in aplastic anemia that are commonly used in the pediatric population include anticonvulsants (hydantoins, carbamazepine ) and carbonic anhydrase inhibitors (acetazolamide and methazolamide ). Many of the drugs listed in Figure 6-1 also have been associated with agranulocytosis. In general only a minority of cases of aplastic anemia can be assigned a drug association. The distinction between aplasia secondary to the medication versus aplasia arising from the underlying disorder (or occult viral infection) requiring treatment can be difficult.
Chemicals and Toxins
Benzene
Benzene is a particularly dangerous environmental contaminant. It is found in organic solvents, coal tar derivatives, and petroleum products. Fatal aplastic anemia, leukemia, or both, have been reported years later in factory workers who had benzene exposure. Benzene is concentrated in bone marrow fat, forms water-soluble intermediates, and damages DNA. It decreases the numbers of progenitors and damages stroma. The risk of cytopenias is likely related to cumulative exposure.
Other Aromatic Hydrocarbons
Toxicity thought to be due to other organic solvents may in some instances be caused by benzene contaminants. Neither pure toluene nor xylene is a marrow toxin. Aplastic anemia has been linked by many case reports to insecticides, particularly γ-hexachlorobenzene (lindane) in children. Aromatic hydrocarbons are present in insecticides and herbicides and may comprise the solvents for these agents. Some organophosphate insecticides have been shown to inhibit in vitro hematopoietic colony formation, as has lindane.
Ionizing Radiation
Marrow aplasia may occur as an acute toxic sequela of irradiation due to nuclear bomb explosion, radioactive fallout, reactor accidents, and accidental exposure in medicine and industry. Bone marrow cells may be affected by high-energy γ-rays as well as by ingested or absorbed lower-energy α particles. The radiation injury is to the actively replicating pool of precursor and progenitor cells and also to stem cells, in which DNA damage may have a more severe effect. Nonetheless, radiation-related marrow aplasia is infrequent. Even in a restricted episode, such as the Chernobyl reactor accident in 1986, most immediate deaths were due to skin burns and damage to gastrointestinal and pulmonary systems.
Chronic radiation-induced aplasia is dose related. Patients who were irradiated for ankylosing spondylitis had an increased risk of aplastic anemia, and American radiologists have been reported to have an increased death rate from aplasia (for both groups, the pathologic distinction of aplasia and myelodysplasia was not made). The incidence of late aplasia in atomic bomb victims was not increased, nor was it increased in patients receiving radiation therapy for malignancies. Knospe and co-workers suggested that irradiation with an exposure greater than 4.4 Gy was required for the development of aplasia; they also postulated that low doses might damage only stem cells, whereas high doses would also damage the supportive hematopoietic stromal microenvironment.
Infectious Agents
Patients with bacterial or viral illnesses frequently develop mild pancytopenia during or following the infection (see Chapter 37 ). Patients with bacterial or viral infections often receive antibiotics and other medications, and it is frequently not clear whether an ensuing aplastic anemia was caused by the infection, by the drug, or by the combination of the two, or even whether the infectious illness was the result and not the cause of the pancytopenia.
Hepatitis
Although hepatitis is frequently associated with mild depression of blood cell counts, aplasia is a rare sequela, estimated to occur in fewer than 0.07% of the total number of pediatric hepatitis cases and in fewer than 2% of those with non-A, non-B hepatitis. Nonetheless as an identifiable clinical event, a prior episode of hepatitis is recognized in 2% to 5% of aplastic anemia patients in a Western series. The prevalence of prior hepatitis is about twofold this proportion in the Far East. Among children with aplastic anemia in Taiwan, 24% had a history of recent acute hepatitis. Antecedent hepatitis may be subclinical, as about 50% of patients with aplastic anemia may have elevated hepatic transaminases before their first transfusion. In a report of 32 patients with liver transplantation for hepatic failure following non-A, non-B hepatitis, 28% developed aplastic anemia. While aplasia has been reported following both hepatitis A and B virus infections, the majority of cases of the hepatitis/aplasia syndrome are not associated with serotypable hepatitis virus.
A study of aplastic anemia patients reported to the European Registry from 1990-2007 found that 5% of patients with aplastic anemia had an antecedent seronegative hepatitis. Hepatitis-associated aplastic anemia was slightly more common in males and tended to present at a younger age. Actuarial survival after treatment with either immunosuppression or hematopoietic stem cell transplant was similar between patients with hepatitis-associated aplastic anemia versus other acquired aplastic anemia.
Flaviviruses
Flaviviruses cause arbovirus hemorrhagic fevers, dengue, and other hematodepressive syndromes. Dengue can propagate in bone marrow cultures without direct cytotoxicity, and dengue antigens induce lymphocyte activation and the release of marrow suppressive cytokines.
Epstein-Barr Virus
Herpesviruses such as Epstein-Barr virus (EBV) are large, complex DNA viruses. EBV causes infectious mononucleosis, which has pancytopenic complications in less than 1% of cases. More than 12 such cases have been reported, and one half of these cases had a fatal outcome. In one study, EBV was demonstrated by immunologic and molecular methods in the bone marrow of six patients with aplastic anemia. Only two had a history of typical mononucleosis, although all six had serologic evidence, suggesting that EBV may be an unrecognized cause of aplastic anemia. The EBV’s target is B cells, although T cells also may be infected. Because EBV is a common infection, issues of ascertainment can render the causative determination of aplasia difficult.
Cytomegalovirus and Human Herpesvirus 6
Infection with cytomegalovirus (CMV) may lead to graft failure in immunosuppressed bone marrow transplant (BMT) recipients. CMV can infect marrow stromal cells in vitro and can inhibit their ability to produce growth factors; direct progenitor cell infection by some CMV strains also has been documented. Herpesvirus 6 is the cause of exanthem subitum and, like CMV, may be found in the marrow of patients with graft failure after transplantation, as well as in hematopoietic progenitors infected in vitro. As with other viruses, both of these are ubiquitous infections making causality difficult to ascertain.
Human Immunodeficiency Virus
Patients with acquired immunodeficiency syndrome (AIDS) often have cytopenias, but their marrow is much more commonly cellular and dysplastic than empty. Colony formation by marrow from patients may be diminished. The action of human immunodeficiency virus-1 (HIV-1), a lentivirus, on hematopoietic cells remains a subject of controversy. HIV-1 infection of CD34+ cells has been difficult to detect in vivo from patient material or after tissue culture infection of normal cells. The virus apparently directly infects megakaryocytes, which bear the CD4 receptor present on T cells. The virus also may affect stroma functions, at least in vitro. HIV-1 can act indirectly on hematopoiesis through inhibitory lymphokine production: The envelope glycoprotein can stimulate macrophages to produce tumor necrosis factor (TNF), which in turn inhibits hematopoietic colony formation. Hematologic suppression can also be due to opportunistic infections, neoplasms, or marrow suppression from the drugs used to treat AIDS and its complications.
Other Viruses
Blood count abnormalities, which are rarely severe, may be observed in the course of rubella, measles, mumps, varicella, and influenza A.
Paroxysmal Nocturnal Hemoglobinuria
Paroxysmal nocturnal hemoglobinuria (PNH) is a disease characterized by variable combinations of mild to severe intravascular hemolysis, large venous thromboses, and aplastic anemia. It is uncommon in adults and even rarer in children (see Chapter 13 ). There is a clear association of PNH with aplastic anemia: Many patients with PNH develop pancytopenia and marrow hypoplasia, and PNH clones are detectable in up to 50% to 70% of patients with acquired aplastic anemia.
PNH is characterized by an inability to inactivate complement on the erythrocyte cell surface, resulting in increased sensitivity to complement. Deficits were subsequently identified in a family of membrane proteins, all of which were anchored to the cell membrane via glycosylphosphatidylinositol (GPI). GPI binds covalently to specific carboxyl terminal protein sequences and attaches them to cell membrane phosphatidylinositol residues. The genetic defect in PNH was localized to the X-linked phosphatidylinositol glycan class A (PIG-A) gene, whose product functions in the transfer of N-acetylglucosamine to phosphatidylinositol as an early step in GPI anchor formation. Early tests for PNH, such as the Ham test or sucrose hemolysis test, relied on the demonstration of increased sensitivity to complement-mediated red cell lysis. Increased sensitivity and specificity are achieved using flow cytometry assays for the absence of GPI-anchored proteins such as CD59 and CD55. More sensitive tests utilize aerolysin, a channel-forming toxin that binds GPI-anchored proteins to result in cell lysis but leaves the GPI-deficient PNH cells intact. The use of a fluorescently labeled aerolysin variant that binds to GPI but fails to lyse the cells offers increased sensitivity and specificity for the detection of the PNH clone.
The clinical significance of small populations of PNH clones is unclear, and most of the published data are derived from adult patients. Small numbers of PNH cells are detectable in healthy controls, although the PNH cells in this context are typically polyclonal. Despite the frequent finding of PNH clones in the bone marrows of aplastic anemia patients, only 10% to 15% of patients subsequently develop the clinical syndrome of PNH. In the majority of patients, such clones may persist or disappear; however, some patients may develop large symptomatic PNH clones requiring therapeutic intervention. Patients with small asymptomatic PNH clones may respond to the same treatments as other patients with aplastic anemia. Patients who develop large PNH clones are at risk for developing symptoms of hemolysis or thrombosis. One recent retrospective study included 47 pediatric patients with aplastic anemia of whom 14 had a PNH clone greater than 1% at baseline (median clone size 16%). The median clone size remained small for all patients, and none of these patients developed clinical symptoms of PNH.
The etiology of marrow aplasia in PNH remains an area of active investigation. Although severe combined immunodeficiency (SCID) mice infused with bone marrow from PNH patients show preferential engraftment with the PNH clones, studies of hematopoiesis in PNH patients have not detected any selective proliferative advantage of the PIG-A − hematopoietic clones. A subsequent study comparing in vitro proliferation of PIG-A − and PIG-A + CD34 cells from PNH patients found a selective growth deficiency in the PIG-A + cell population rather than an advantage for the PIG-A mutant cells: Fas expression was elevated on the wild type compared with the GPI-deficient cells, suggesting increased resistance to apoptosis as one potential mechanism to explain their findings. No proliferative advantage of PNH hematopoietic clones was observed in mice mosaic for the PIG-A gene. To evaluate the hypothesis that autoreactive T cells might preferentially eliminate PIG-A + hematopoietic stem cells while sparing the PNH clones, the sensitivity of normal versus PNH EBV-transformed B-cell lines to autologous EBV-specific T-cell lines was examined. The PNH cells were no less sensitive to T-cell-mediated cytotoxicity than the non-PNH cells; thus the GPI-linked cell surface molecules are not required for killing by T cells. An abnormal distribution of expanded T-cell clones detected by size analysis of the complementarity-determining region 3 (CDR3) in the β-variable region (BV) mRNA of the T-cell receptor (TCR) has been noted in PNH patients, although the targets of such T-cell populations remain to be ascertained. The mechanisms underlying the clonal expansion of the GPI-negative cells in PNH or aplastic anemia is currently unclear. Despite the presence of clonal populations of PNH cells, patients with PNH do not exhibit an increased incidence of leukemias, and the GPI-negative clones do not behave in a malignant fashion.
Immunologic Diseases
Ten percent of patients with the rare collagen vascular syndrome, eosinophilic fasciitis, have associated aplastic anemia. This condition is usually limited to adults. Thymoma associated with hematopoietic failure generally but not exclusively presents as pure red cell aplasia. Some of the adult cases had also received drugs such as chloramphenicol or sulfonamides, and the aplasia could have been due to the drugs alone or to the combination. An iatrogenic aplasia can be induced by the transfusion of competent lymphocytes into immunodeficient hosts. In these cases, marrow failure arises from the transfusional graft-versus-host disease (GVHD).
Pathophysiology
The purported mechanisms for failure of hematopoiesis are predicated on our current knowledge of normal hematopoiesis (see Chapter 7 ). Disease could result from decreased numbers or defective function of the cellular or soluble components required for blood cell production or from exogenous factors that result in damage or elimination of hematopoietic cells. Figure 6-2 depicts different potential mechanisms for the loss of hematopoietic cells in aplastic anemia. In model I, marrow toxins such as irradiation, drugs, or chemicals or direct invasion by viruses might cause hematopoietic stem and/or progenitor cell death. In model II, an underlying abnormality of the hematopoietic cells may result in a predisposition to hematopoietic stem cell damage, premature stem cell loss, or insufficient stem cell production (see Chapter 1 ). In model III, viruses, drugs, toxins, or immune dysregulation may incite a cellular or humoral immunologic reaction against a normal hematopoietic compartment. In model IV, abnormalities of the marrow stromal environment may actively inhibit hematopoiesis. These possibilities are not mutually exclusive, and supportive data for each model have been described. Current data suggest that aplastic anemia may represent the final seemingly common outcome of different pathogenic mechanisms, with different underlying causes entailing different medical management considerations.
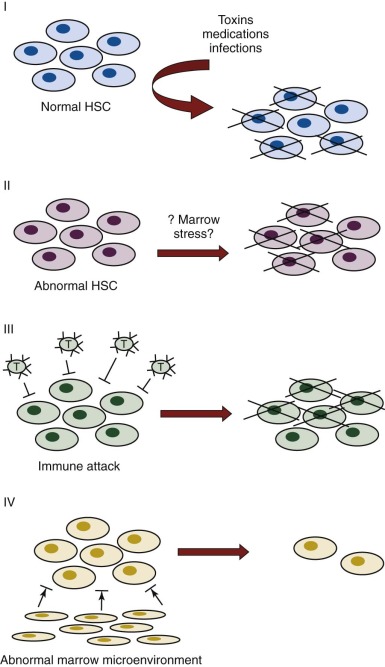
Hematopoietic Stem Cells and Clonality
Patients with aplastic anemia have decreased numbers of blood and marrow committed progenitor cells, assayed as myeloid colony-forming cells (CFU-GMs); erythroid colony-forming cells (CFU-Es and burst-forming units erthyroid [BFU-Es]); megakaryocytic progenitors (CFU-megas); multipotent colony-forming cells (CFU granulocyte, erythrocyte, monocyte, megakaryocyte [CFU-GEMMs]); and long-term culture-initiating cells (LTC-ICs). Hematopoietic cells can also be assessed phenotypically for the presence of the CD34 antigen, which defines a compartment of about 1% of marrow cells that includes progenitors and possibly activated stem cells. CD34+ cells are reduced in aplastic anemia, and almost all patients show not only severe reduction in the numbers of these cells but also poor plating efficiency for colony formation from purified CD34+ cells.
Several lines of evidence point to primary abnormalities in the hematopoietic stem cell as a potential etiology for aplastic anemia. Multiple studies have noted that simple infusion of stem cells from an identical twin donor without prior conditioning was sufficient to treat the aplasia in many cases. These results strongly support a pathogenic stem cell defect and argue against permanent disorders of the bone marrow environment, including immunologic mechanisms, as the cause of stem cell destruction. Low stem cell numbers persist even with hematologic recovery after medical therapy with antithymocyte globulin (ATG) and cyclosporine (see following discussion), and blood cell parameters, such as macrocytosis, do not return to normal in many cases, again suggestive of an underlying stem cell abnormality. A primary stem cell defect likely also contributes to the observation of clonal hematopoiesis in aplastic anemia as well as late development of other hematologic diseases such as myelodysplasia and acute leukemia (see following discussion).
A subset of patients with seemingly idiopathic aplastic anemia may actually carry an inherited/congenital predisposition to marrow failure (see Chapter 7 ). Although the inherited/congenital marrow failure syndromes are often associated with characteristic physical findings, patients may present with isolated aplastic anemia as the sole manifestation of their underlying inherited syndrome. In some patients lacking significant physical findings or cytopenias at the time of evaluation, an elevated mean corpuscular volume (MCV) may be the only clue to an underlying inherited marrow failure syndrome. A careful family history for hematologic abnormalities, malignancy predisposition, or other characteristic clinical stigmata may be helpful in distinguishing an inherited marrow failure syndrome. For example a subset of patients presenting with aplastic anemia without other clinical stigmata of an inherited marrow failure syndrome were found to harbor mutations in the dyskeratosis congenita genes hTERC, which encodes the RNA component of telomerase, or hTERT, which encodes the catalytic subunit of the telomerase complex. Telomeres are specialized structures that stabilize the ends of each chromosome to prevent excessive shortening with replication, to distinguish chromosome ends from internal DNA breaks, and to prevent end-to-end fusions. Telomeres consist of six–base pair repeated sequences (TTAGGG) associated with a specific protein complex. Telomerase is a ribonucleoprotein enzyme consisting of an H/ACA RNA component (TERC) complexed with a reverse transcriptase (TERT) and additional H/ACA binding proteins including dyskerin, GAR1, NHP2, and NOP10. When telomerase levels are diminished or absent, telomeres progressively shorten with each cell division. At a critically short telomere length, a checkpoint is triggered for cells to stop dividing and undergo senescence to prevent chromosomal rearrangements.
Shortened telomeres have been observed in the leukocytes of aplastic anemia patients. Of five patients noted to have mean telomere lengths less than 5 kb, three had acquired cytogenetic abnormalities, suggesting that compromise in the telomere’s probable role in stabilizing chromosome ends may contribute to the increased incidence of myelodysplasias and acute leukemias. A study by Brummendorf and colleagues reported relatively normal telomere lengths in patients who responded to immunosuppression, while untreated or unresponsive patients showed significant telomere shortening. Telomere shortening is a common feature associated with marrow failure, though telomere lengths are particularly short in patients with dyskeratosis congenita, a disorder of telomerase function, even in comparison to other marrow failure patients. Whether telomere shortening in marrow failure patients lacking telomerase mutations represents a primary defect in telomere maintenance or a secondary effect of increased cycling of the few remaining hematopoietic stem cells remains to be clarified. Shorter telomere lengths have been associated with a higher risk of relapse and clonal progression with decreased survival in patients with aplastic anemia.
Immune Destruction
Several lines of data suggest that immune phenomena may result in aplastic anemia. In some syngeneic (identical twin) BMTs, simple infusion of stem cells is not sufficient to reconstitute hematopoiesis. The requirement for prior conditioning of the host may reflect some operational immunologic process, though it is also consistent with the presence of an underlying clonal abnormality that requires ablation. Histocompatibility studies conducted in Europe, Asia, and America have indicated an increased representation of HLA-DR2 among aplastic anemia patients, consistent with a genetically determined immune susceptibility to disease. Evidence for specific immune mechanisms is reviewed below.
Lymphocytes
Lymphocyte infusions in mouse models result in marrow destruction and aplastic anemia. Autologous recovery has been reported after either mismatched or matched transplants in patients prepared with antilymphocyte sera or cyclophosphamide. While the etiology of aplastic anemia in children remains unclear, evidence supports an immunologic mechanism of disease in the absence of known environmental toxins, drugs, and constitutional syndromes. Overriding these considerations are the large proportion of responses in acquired aplastic anemia to immunosuppressive therapies, although the antilymphocyte preparations contain heterogeneous mixtures of antibodies to many cells, including lymphocytes , and are clearly immunosuppressive as well as generally cytotoxic. Cyclosporine exerts inhibitory T cells effects and inhibits transcription of genes for cytokines including IL-2 and interferon-gamma (IFN-γ). It has many other toxic effects on several tissues, including brain and kidney. The diverse antibodies in ATG react with a wide range of antigens, including signal transduction and adhesion molecules. Thus the attribution of immune dysregulation as causal in aplastic anemia based on therapy must be tempered with caution because ATG and cyclosporine exert multiple effects in addition to immunosuppression. Of note, therapies with mixtures of monoclonal antibodies specific for human T cells have not been effective in clinical trials to date (see following discussion). Furthermore ATG can increase colony growth in normal, myelodysplastic syndrome (MDS), and aplastic anemia CD34+ bone marrow cells in culture. ATG treatment also reduces the expression of Fas-Ag on aplastic anemia bone marrow CD34+ cells. Effective treatment of myelodysplasia with immunosuppressive medications has been reported and may suggest that such agents might exert additional effects on the bone marrow.
Nonetheless multiple reports have demonstrated an association of aplastic anemia with alterations in lymphocyte numbers or specific immunologic changes at presentation and in some cases further changes with hematologic responses. Hoffman and colleagues showed inhibition of erythroid growth in vitro utilizing coculture of peripheral blood lymphocytes from five out of seven aplastic anemia patients studied. Zoumbos and colleagues noted increased circulating and bone marrow levels of IFN-γ in aplastic anemia patients and a response of increased colony growth in vitro in response to inhibition with neutralizing antibody to this proinflammatory cytokine. Hinterberger and colleagues failed to confirm the reported increased circulating levels of proinflammatory cytokines in aplastic anemia patients, but did demonstrate increased TNF and IFN-γ production by peripheral blood mononuclear cells (PBMCs) from aplastic anemia patients at baseline and after stimulation in vitro. Risitano and colleagues reported a dominant clonal T-cell immune response characterized by flow cytometry using antibodies against different TCR-β subfamilies and by sequencing the CDR3 region of the TCR-β chain in 54 patients studied with aplastic anemia. The size of these clones and the total number of T cells decreased following successful treatment with ATG and increased with relapse. This phenomenon was associated with HLA-DR1501 genotype and activated cytotoxic T cells assayed in vitro. The association with HLA type was not confirmed by a subsequent study of Japanese patients. Solomou and colleagues reported reduced T regulatory cells (TRegs) (CD4+, CD25+, FoxP3 + ) in 20 patients with aplastic anemia that was consistent with increased IFN-γ and a TH1 polarization phenotype of T cells. Six of seven patents showed slightly increased numbers of TRegs after immunosuppression therapy (IST) during hematologic responses. In a case report, Ikeda and colleagues noted marked alteration of the CD4/CD8 T-cell ratio after hepatitis that was subsequently followed by onset of aplastic anemia. IST was followed by normalization of lymphocyte subsets with hematologic recovery.
These studies imply concomitant immune dysregulation with aplastic anemia and suggest a mechanism by which IST could be working therapeutically. However, no specific antigen has previously been implicated mechanistically in the evolution of aplasia. In a recent report, it was shown that a prodrug of the anti-HIV drug, abacavir, which is associated with a hypersensitivity reaction in treated patients, can bind to the HLA-B*57:01 molecule, resulting in altered peptide presentation. The altered peptides appear as foreign and lead to activation of CD8+ T cells. One can imagine such a scenario leading to acquired aplastic anemia if the abnormal peptide presentation of “self-antigens” leads to CD8 activation directed against hematopoietic stem cells in the bone marrow. While such a finding is not reported yet in aplastic anemia patients, this could underlie the immunologic mechanism long hypothesized as the etiology of this disease. Interestingly Weinstock and colleagues also recently demonstrated somatic alterations in 20% of adult patients with acquired aplastic anemia utilizing next generation sequencing technology. Alterations included mutations of DNMT3A and ASCL1, both associated with MDS in adults, and mutations of β 2 -microglobulin, which is required for class I expression and CD8 T-cell function. Thus previously unrecognized mutations may predispose to acquired aplastic anemia via either mechanisms leading to a prodrome of myelodysplasia or by subtle alternations in immune functions. Limitations of these studies include the paucity of purified cell populations required for sequencing and the lack of functional data that addresses the biologic relevance of these alterations with respect to stem cell function and hematopoiesis. Additional studies will undoubtedly follow.
Lymphokines
Whether overproduction or dysregulated production of inhibitory cytokines represents a primary etiology versus a secondary effect of an underlying bone marrow abnormality remains unclear. IFN-γ, a soluble inhibitor produced by T cells, was found to be produced at high levels in cultures from aplastic anemia patients. IFN-γ is expressed in the marrow of most patients with aplastic anemia but not in normal people or in patients with other hematologic diseases who have undergone frequent transfusions. High pretreatment intracellular γ-interferon levels correlated with response to immunosuppressive therapy. Other inhibitory cytokines, such as TNF and macrophage inflammatory protein-1, also are overexpressed in aplastic marrow. Both interferon and TNF directly and synergistically inhibit hematopoiesis in vitro. In long-term bone marrow culture, constitutive low-level expression of IFN-γ is sufficient to markedly reduce the output of committed progenitor cells and LTC-ICs, the stem cell surrogate. Both interferon and TNF increase the potential for programmed cell death within the CD34+ compartment by increasing Fas antigen expression on target cells. Fas antigen is a cell surface molecule in the TNF receptor family; its activation signals apoptosis.
Microenvironment
In theory aplastic anemia could be due to a microenvironment that fails to support hematopoiesis: a lesion of “soil” rather than “seed.” The role of the HM in etiology of aplastic anemia or the response to treatment remains controversial. Ershler and colleagues showed decreased erythroid growth in whole bone explants from a single patient with constitutional red cell aplasia but no effect from the patient’s plasma, mononuclear cells, or T cells, implying an HM defect. This observation is reminiscent of the HM defect seen in Steel mice, which lack production of normal stem cell factor (SCF) in nonhematopoietic cells, but it seems more likely that this was simply a demonstration of a cell-intrinsic erythroid cell defect. Dexter and colleagues described an in vitro long-term marrow culture (LTMC) that consisted of an adherent cell compartment made up of a number of different cell types that supported hematopoiesis for weeks to months ex vivo. Subsequently Marsh and colleagues examined LTMC from 32 adult aplastic anemia patients and found 31 of the 32 had abnormal hematopoiesis in these cultures. However, using cross-over cultures, only 1 of 23 patients had defective adherent components. Juneja and colleagues demonstrated reduced cobblestone areas (containing active hematopoiesis) but normal adherent cell fractions in eight aplastic anemia patients compared with normal controls. Marsh and colleagues utilized human LTMC and assessed the HM in cross-over experiments. They demonstrated that stroma generated from aplastic anemia patients performed normally when inoculated with CD34+ cells from normal donors. Nissen and colleagues compared short-term stroma and progenitor colony growth in aplastic anemia patients treated with or without IST and found a modest increase in both of these cell fractions in treated versus untreated patients. Chatterejee and colleagues used a cytotoxic drug animal model of aplasia to demonstrate failure of recovery of marrow stromal cells with time. While this study may be relevant to some isolated instances of acquired aplastic anemia, it seems more relevant to marrow damage in cancer or leukemia patients undergoing therapy. Shipounova and colleagues showed impaired adipogenesis and osteogenic potential with increased size and number of fibroblast colonies from aplastic anemia patients’ bone marrow. These authors also demonstrated increased stromal-derived cytokines, including ANG1, VEGF, and VCAM-1. Overall these studies suggest some alterations in the HM of aplastic anemia patients, but there is currently no definitive evidence in humans of a mechanistic link between defective marrow microenvironment and development of acquired aplastic anemia.
More recently interest has focused on defining specific anatomic and/or biochemical spaces (niches) in the bone marrow occupied by hematopoietic stem cells. Schofield first termed the phrase stem cell niche to define a supporting cellular environment in which hematopoietic stem cells reside. Currently controversy exists about the overall relevance of the two best-studied stem cell niches. Hematopoietic stem cells appear to reside in close proximity to osteoblasts that line the cortical bone of the marrow space, and osteoblasts may be important in maintaining quiescence of the primitive hematopoietic stem cells. This area of the marrow has thus been termed the osteoblastic niche. A subset of these cells, spindle-shaped N-cadherin + CD45 − osteoblasts (SNO cells) residing in trabecular bone, may be derived from mesenchymal stem cells, and their number correlate with the content of long-term hematopoietic stem cells . Ablation of osteoblasts leads to loss of HSC and failure of hematopoiesis, while manipulations that increase osteoblasts have the opposite effect.
An anatomically distinct putative hematopoietic stem cell niche is made up in part by sinusoidal endothelial cells and has been termed the perivascular niche. Some data suggest this niche may be critical for mobilization and homing of hematopoietic stem cells via the Rac GTPase pathway and/or for progenitor cell proliferation and differentiation, although these studies have not examined long-term hematopoietic stem cells in functional assays. Alterations of the sinusoidal endothelium with antibodies to VE-cadherin leads to failure of megakaryocytopoiesis in murine models. Some models suggest that quiescent hematopoietic stem cells may migrate from the osteoblastic niche to the perivascular niche via β1 integrin function and chemokine/growth factor stimulation. Overall, whether there are truly distinct functional differences between these putative hematopoietic stem cell niches remains to be determined, and the role of specific growth factors, adhesion molecules, and chemokines are incompletely understood. A long-term goal of investigators in this field is to recapitulate the HM in vitro such that true expansion of hematopoietic stem cells may occur in the laboratory setting. Success in this area would have a major impact in BMT and regenerative medicine.
Hematopoietic growth factor production and plasma levels are usually increased rather than decreased in patients with aplastic anemia. Circulating levels of erythropoietin, granulocyte colony-stimulating factor (G-CSF), and granulocyte-macrophage colony-stimulating factor (GM-CSF), thrombopoietin, and flt-3 ligand are elevated in patients with aplastic anemia. SCF levels have been reported to be low to normal. Levels of interleukin 1 (IL-1), produced by monocytes, may be low in aplastic anemia. Therapeutic trials with these factors have yielded divergent and incomplete responses, casting doubt on the pathophysiologic significance of deficiency of these factors. The report of inhibitory effects of marrow adipocytes on hematopoiesis in mouse models is intriguing given the typical fatty replacement observed in aplastic bone marrows.
Clinical Evaluation
The differential diagnosis of pancytopenia is broad ( Box 6-3 ). The evaluation and diagnostic work up of the patient with presumed acquired aplastic anemia should be directed at eliminating alternative diagnoses for which curative therapies are available or for which the therapies for aplastic anemia would be inappropriate ( Table 6-1 ). Because the inherited marrow failure syndromes entail different medical management issues, a careful medical history, family history, physical examination, and appropriate laboratory evaluation to assess for underlying inherited bone marrow failure syndromes should be pursued. The diagnosis of an inherited marrow failure syndrome also carries profound implications for hematopoietic stem cell donor selection from family members and may be important for family planning. Given the wide clinical variability of the inherited marrow failure syndromes even amongst members of a given family, all siblings of a patient with an inherited marrow failure disorder should be tested whether or not they appear to carry clinical stigmata of the disease.
Hypocellular Bone Marrow
Acquired aplastic anemia
Inherited bone marrow failure syndrome
(Fanconi anemia, amegakaryocytic thrombocytopenia, dyskeratosis congenita, Shwachman–Diamond syndrome, GATA-2 syndromes)
Paroxysmal nocturnal hemoglobinuria*
Hypoplastic myelodysplastic syndrome
Virus-associated aplastic anemia
Cellular Bone Marrow
Primary Bone Marrow Disease
Myelodysplasia
Paroxysmal nocturnal hemoglobinuria*
Secondary to Systemic Disease
Systemic lupus erythematosis, Sjögren syndrome
Hypersplenism
Vitamin B 12 , folate deficiency
Infection
Storage disease (Gaucher, Niemann-Pick)
Alcoholism
Sarcoidosis
Infiltrative bone marrow disorders
Acute myelogenous leukemia
Acute lymphoblastic leukemia
Hemophagocytic lymphohistiocytosis
Metastatic solid tumors
Osteopetrosis
Myelofibrosis
Clinical History | Rationale |
Recent illnesses | Infectious etiology |
Medications | Marrow suppression |
Toxic exposures | Marrow suppression |
Family history of blood disorders, malignancy, congenital anomalies | Assess for inherited marrow failure |
Physical Examination | |
Vital signs | Clinical sequelae of cytopenias |
Height and weight | Assess for inherited marrow failure |
Congenital anomalies, stigmata | Assess for inherited marrow failure |
Assess for other systemic diseases | |
Laboratory Tests | |
Complete blood count and differential | Defines severity |
Morphology | Malignant versus benign |
Vitamin B 12 deficiency | |
Storage disease | |
Reticulocyte count | Defines severity |
Differentiate production versus destruction | |
Bone marrow biopsy | Assess cellularity |
Assess architecture (granuloma, fibrosis, hemophagocytosis, infiltrative or metastatic disease) | |
Bone marrow aspirate | |
Morphology | Malignant versus benign |
Storage disease | |
Hemophagocytosis | |
Congenital disorder | |
Cytogenetics | Myelodysplasia |
Culture | Infectious agent (e.g., tuberculosis, virus) |
Other | DNA/antigen-based viral tests |
Peripheral blood | |
Chemistry | |
AST, ALT, GGT, Bilirubin, LDH | Hepatitis |
Coombs test | Autoimmune cytopenias |
BUN, creatinine, electrolytes | Chronic renal failure |
Serologic testing/PCR | Hepatitis, EBV, HIV, other virus |
Flow cytometry for CD55/CD59 | Paroxysmal nocturnal hemoglobinura |
Diepoxybutane or mitomycin C chromosomal breakage | Fanconi anemia |
Telomere length, dyskeratosis congenita | |
Autoimmune disease evaluation | Evidence of collagen vascular disease |
Histocompatibility testing of patient and family | Establish potential donor pool |
History
The chief presenting symptoms relate to low blood counts. Bleeding, such as gum oozing, nosebleeds, easy bruising with minimal trauma, or heavy menses may be seen. Chronic anemia may present with fatigue, decreased activity, or exercise intolerance, though the gradual onset of severe anemia can be surprisingly well compensated in young children. Serious infection is not a frequent presenting symptom early in the course of aplastic anemia. Many patients feel well with few symptoms on initial presentation. A history of steatorrhea or fatty food intolerance may be a manifestation of Shwachman–Diamond syndrome, though the absence of these symptoms does not rule out this diagnosis. Gastrointestinal disorders may also be associated with other inherited marrow failures syndromes such as dyskeratosis congenita or Fanconi anemia. A careful family history for blood disorders, malignancies (particularly ovarian and breast cancer or hepatocellular carcinoma), premature graying, hepatitis, pulmonary fibrosis, or congenital anomalies that might suggest an inherited bone marrow syndrome should be sought. Patients with a history suggestive of a familial marrow failure syndrome or familial MDS should be evaluated further with genetic and laboratory studies. A developmental history can provide a helpful screen for potential congenital, metabolic, or storage diseases. A detailed medication, environmental, and infectious history, particularly for the period 1 to 12 months prior to presentation, should be obtained.
Physical Examination
Clinical appearance is related to the severity and duration of the underlying pancytopenia. Hemorrhagic manifestations from thrombocytopenia occur early and include petechiae (particularly on the border of the hard and soft palate), ecchymoses, epistaxis, and bleeding from mucous membranes. Neutropenia may be associated with oral ulcerations, bacterial infections, and fever; these signs are rarely present early. Evidence of erythropoietic failure characterized by pallor, fatigue, and tachycardia is often late because the life span of the erythrocyte (120 days) far exceeds that of platelets (10 days) or white cells (variable, but measured in hours for granulocytes). Mucous membranes and nail beds may be pale. Lymphadenopathy, splenomegaly, and severe weight loss are uncommon and may suggest other underlying disorders. Short stature, congenital anomalies (particularly of the thumbs and forearms), areas of hyper- or hypopigmentation, dystrophic nails, immunologic abnormalities, or pulmonary disease should alert the examiner to possible inherited bone marrow failure disorders. Immunologic abnormalities or lymphedema raises consideration of inherited GATA-2 syndromes (see Chapter 24 ).
Laboratory Studies
Evaluation should include a complete blood count and reticulocyte count. A careful evaluation of the peripheral blood smear may suggest infection or dietary deficiency. Blood counts are most often uniformly depressed. The blood smear shows a paucity of platelets and leukocytes. The anemia is often macrocytic and particularly useful in pointing to a possible constitutional syndrome, though if erythropoiesis is entirely arrested, it may be normocytic. The red cell distribution width (RDW), a numeric measure of anisocytosis, is most often normal. Platelet size is not increased, as it is in most cases of immune thrombocytopenias. The absolute reticulocyte count is decreased. Granulocyte numbers are clearly diminished, as may be those of monocytes and lymphocytes. Increases in fetal hemoglobin (Hb F) and red cell i antigen are manifestations of the fetal-like erythropoiesis seen in stress hematopoiesis but occur irregularly in aplastic anemia patients, have no prognostic value, and can persist in recovered patients.
Laboratory evaluation for possible infectious etiologies should be obtained as clinically indicated. Additional testing for possible immunologic or autoimmune diseases may be considered. Blood tests to rule out Fanconi anemia and PNH are important, as these diagnoses would entail alternative treatments. Telomere length measurements may be useful as a screen for possible underlying telomere disorders. Very short telomeres in long-lived lymphocyte populations should prompt consideration of additional genetic workup for dyskeratosis congenita. Data on the use of telomere measurements in isolation to guide clinical treatment decisions in this setting are sparse. A growing array of genetic testing is available to assist in the diagnosis of many of the inherited marrow failure syndromes (see Chapter 7 ). Patients with stigmata of collagen vascular disease should be appropriately evaluated. Early HLA typing of the patient and family members is helpful in guiding future therapies. Baseline renal and hepatic laboratory evaluations are also indicated prior to the initiation of potentially nephrotoxic or hepatotoxic therapies.
Bone marrow examination must be done by both aspiration and biopsy so that cellularity can be evaluated both qualitatively and quantitatively. The bone marrow in aplastic anemia is hypocellular, with empty spicules, and increased fat, reticulum cells, plasma cells, and mast cells. In young children there may be residual lymphocytes that appear prominent due to the lack of myeloid elements in the marrow cavity. Some dyserythropoiesis, with megaloblastosis and nuclear-cytoplasmic asynchrony, may be seen. Aspirates alone may appear hypocellular owing to dilution with peripheral blood, or they may look hypercellular because of areas of focal residual hematopoiesis. Biopsies provide more reliable specimens for assessment of cellularity. Biopsies also provide critical information regarding bone marrow architecture such as fibrosis or granulomas. Bone marrow aspirates should also be sent for karyotype analysis and fluorescence in situ hybridization (FISH) to assess for clonal cytogenetic abnormalities associated with myelodysplasia or malignancy. While FISH studies are more sensitive, they can only detect the specific chromosomal abnormality tested. Bone marrow may also be sent for culture or DNA-based antigen detection of infectious agents such as viruses, though interpretation is tempered by the frequent persistence of viral DNA long after the active infection has passed.
Management and Outcome
Prognosis
Outcome depends on the types of treatments offered, the causes of the aplasias, and the eras and countries being analyzed. In a large series conducted before 1957, Wolff found a survival rate of only 3%. In other series ending no later than 1965, complete recoveries were reported in 10% to 35% of patients. In a series of 40 pediatric patients seen before 1958, Shahidi and Diamond found that only two patients recovered, one of whom later had recurrence, though these studies may have included patients with moderate disease for whom spontaneous recovery is more common. Long-term prognosis is adversely affected by delayed complications such as clonal disease. The clinical course of 24 pediatric patients with moderate aplastic anemia was followed for a median of 66 months (range 10 to 293 months). Moderate aplastic anemia was defined as marrow hypoplasia of less than 50%, at least two cytopenias such as an absolute neutrophil count (ANC) less than 1500/mm 3 , platelet count less than 100,000/mm 3 , or reticulocytopenic anemia lasting at least 6 weeks. Sixteen patients (67%) progressed to severe aplastic anemia, five patients (21%) remained in the moderate range, and three patients (12%) resolved. It is currently unclear whether early institution of therapy for moderate aplastic anemia confers improved outcome. Because current therapies are associated with significant side effects, their institution for children with moderate aplastic anemia, for whom the marrow aplasia may spontaneously resolve, warrants careful consideration.
Spontaneous Recovery
Spontaneous recovery has been reported anecdotally, although most cases were probably of moderate rather than of severe aplastic anemia. Good supportive care (see following discussion) may contribute to this; in one series, 14 of 33 children recovered, and children with moderate aplastic anemia have been found to have an excellent prognosis even without specific treatment. A study of immunosuppressive therapy in which 21 patients were randomized (some to receive only supportive care) found no improvement in the control group after 3 months. Spontaneous recovery is sufficiently rare in severe aplastic anemia that all modalities of current therapy should be initiated.
Supportive Care
Blood product support should be used judiciously to avoid sensitization, and blood product donors should never be from the patient’s family for the same reason. Complete red cell phenotyping should be considered where feasible to minimize the potential risk of erythrocyte antibodies in patients who may receive long-term blood product support. All blood products should be irradiated because standard treatments are associated with profound immunosuppression. Leukoreduction appears prudent to reduce the potential risk of infection transmission and allosensitization because aplastic anemia patients may require a hematopoietic stem cell transplant. Exposure to potentially hazardous drugs or toxins should be eliminated. Treatment with single hematopoietic growth factors, such as granulocyte-colony stimulating factor (G-CSF) or erythropoietin should be avoided in part due to the resulting delay in definitive therapy.
Bleeding
Platelet (and red cell) support has probably had the greatest impact on survival in aplastic anemia and has changed the cause of death from bleeding to infection. Platelets can be provided from several individual units of blood or, preferably, by platelet pheresis from a single donor to reduce antigenic exposure (see Chapter 36 ).
The main role of prophylactic platelet support is reduction of the risk of intracranial hemorrhage, which is a rare but potentially devastating event. The general threshold platelet count has traditionally been 20,000 cells/µL; however, this figure and the entire practice of prophylactic administration of platelet transfusions have recently been questioned. Platelet transfusion at platelet counts below 10,000/µL in stable outpatients with chronic severe aplastic anemia was feasible and safe. Chronic platelet transfusions for aplastic anemia are generally given when there are symptoms of bleeding or if the patient is at increased bleeding risk (e.g., toddlers learning to walk, patients with hypertension while on cyclosporine, patients with fever and infection). In some institutions a higher platelet transfusion trigger point of 20,000/µL is used during initiation of IST.
Other measures to reduce bleeding include maintenance of good dental hygiene, use of a soft toothbrush, stool softeners, and avoidance of trauma. Drugs that may interfere with platelet function, such as aspirin or nonsteroidal antiinflammatory drugs, should be avoided in thrombocytopenia. Antifibrinolytic agents such as aminocaproic acid and tranexamic acid may decrease bleeding, particularly from the gums and nasal mucosa.
Infections.
There are few reported studies of infection in aplastic anemia. Severe granulocytopenia may last for years, and infection remains the leading cause of death in aplastic anemia patients, particularly bacterial and fungal infections. However, the immune systems of granulocytopenic patients remain intact; this is in contrast to the situation of patients with malignancies who receive chemotherapy and who experience simultaneous neutropenia and immunosuppression (as during IST for aplastic anemia). Neutropenia (and perhaps monocytopenia) increases the risk of bacterial infection in aplastic anemia. Because neutropenia precludes the development of an inflammatory response, identification of an infected locus is often difficult. The bacterial organisms may be gram-negative bacilli such as Escherichia coli, Klebsiella pneumoniae, and Pseudomonas aeruginosa, as well as gram-positive cocci, including Staphylococcus aureus and S. epidermidis and streptococci. Use of G-CSF has been studied in a few prospective analysis and other randomized trials, and these studies have been examined by meta-analysis. Gluckman and colleagues randomized aplastic patients receiving IST to prophylactic G-CSF treatment or not. These investigators reported enhanced recovery of neutrophils in the G-CSF group but no effect on overall survival. More recently, Tichelli and colleagues randomized 192 aplastic patients to ATG/cyclosporine +/− G-CSF and reported significantly fewer infectious episodes and hospitalization days in the G-CSF group but no differences in overall survival or event-free survival between groups. Interestingly they found that the lack of neutrophil response at day 30 post initiation of treatment was significantly associated with a lower response rate overall and a lower survival rate. They postulated that if confirmed by additional studies, the lack of neutrophil recovery at 30 days posttreatment could identify a group of patients in which alternative treatment might begin earlier than in the past. This study did not confirm a prior study by Teramura and colleagues that reported a lower incidence of relapse in patients treated with G-CSF. Meta-analysis of a large number of studies has also reported that in aggregrate these studies showed no effect of G-CSF on overall survival, hematologic responses, or specific infections and no association of the use of G-CSF with MDS, AML or PNH.
Immunosuppression due to preparation for BMT or as primary therapy for the aplastic anemia may lead to unusual bacterial, fungal, viral, and protozoan infections. Recommendations for specific antibiotics and other antiinfection agents are beyond the scope of this chapter, and regimens are changing rapidly as new generations of treatments are developed.
The use of routine prophylactic antibiotics has no demonstrated role in the “well” patient with aplastic anemia. In many institutions, guidelines are derived from experience in neutropenic cancer and post–BMT patients because prospective studies in pediatric aplastic anemia patients are rare. In the opinion of many, even without supporting outcomes data, aplastic anemia patients with ANC less than 500 should receive antibacterial and antifungal prophylaxis. Patients undergoing IST are typically given prophylaxis for Pneumocystis carinii , although data supporting the need for this treatment are sparse or nonexistent. In the context of fever and neutropenia, complete evaluation and cultures of all possible sites should be followed by the administration of broad-spectrum parenteral antibiotics. Fungal infections occur frequently in neutropenic patients who have received repeated or extended courses of antibiotics; candidiasis and especially aspergillosis, which lead to sinusitis, lung disease, or disseminated infection, are distressingly frequent causes of death in aplastic anemia. Antecedent or concurrent administration of steroids increases this risk. Aggressive introduction of antifungal treatment is indicated in the persistently febrile patient who is unresponsive to antibiotics or in the appropriate clinical setting.
Anemia.
Red cells should be provided as clinically indicated. The hemoglobin should be maintained at a level consistent with normal activities if routine transfusions are to be used. Chronic anemia can be well tolerated once adaptation has occurred, and some children who can sustain a hemoglobin level greater than 6 to 7 g/dL without transfusions (i.e., a child who is not bleeding) may be carefully observed. Long-term transfusions lead to iron overload, with accumulation in critical organs. Permanent damage to heart, liver, and endocrine glands from iron overload may be prevented by iron chelation therapy.
Treatment
Hematopoietic Stem Cell Transplantation.
Hematopoietic stem-cell transplant offers curative therapy for aplastic anemia. The general topic of BMT is described in Chapter 8 . The role of BMT in aplastic anemia treatment is discussed in Chapter 8 .
Immunosuppression
Antithymocyte Globulin.
Antilymphocyte globulin (ALG) and ATG (the sera produced by immunization of horses or rabbits with human thoracic duct lymphocytes or thymocytes) are highly cytotoxic reagents with activity against all blood and marrow cells, including progenitors. These reagents were initially used to decrease the rate of rejection of HLA-matched bone marrow. ALG and/or cyclophosphamide were also employed to permit at least temporary engraftment with mismatched marrow in aplastic patients without a matched donor. Autologous recoveries occurred in some of these patients; they encouraged several groups to examine the use of ATG or ALG with and without haploidentical marrow. Treatment with ALG was superior to controls in both response rate and 3-year survival. The addition of additional agents to ALG therapy can further enhance therapeutic effect.
ATG or ALG/ALS is often given to a total cumulative dose of 100 to 160 mg/kg, typically divided over 4 days. Immediate allergic reactions to ALG are rare and can be predicted by skin testing followed by desensitization in those who are allergic. The patient begins to make antibodies to horse protein about 1 week after first exposure; at this point, equine antibodies are rapidly cleared from the circulation. This reduces the effective dose and enhances immune complex formation. Serum sickness due to immune complex deposition may manifest around 11 days after initiation of treatment as fever, a serpiginous rash at the volar–dorsal border of the hands and feet, arthralgia, myalgia, lassitude, and changes in urine sediment. Bronchospasm or liver chemistry abnormalities may also occur. A short course of corticosteroids is usually given (prednisone, 1 mg/kg) beginning at the start of ATG treatment and for 10 to 14 days to reduce ATG-related side effects. ATG binds to determinants on all cell types in blood and marrow and reduces platelet and granulocyte counts in addition to the lymphocyte counts, and it may lead to a positive Coombs test. Thrombocytopenia may require intensive blood product support during this time.
Responses to ATG given as a single agent usually occur within the first 3-months, if at all, and blood count improvement by 3 months correlates with survival. The earliest response to therapy may be heralded by the appearance of a few granulocytes and nucleated red cells in the circulation. Red-cell size distribution histograms typically show a macrocytic shoulder. Reticulocytes appear, transfusions decline, and the hemoglobin level increases slowly. The white cell count rises next. The last cell line to return is often the platelets, whose numbers may remain low for months or even years. The recovering red cells are not normal; in addition to macrocytosis, Hb F level is increased, and fetal membrane antigens may remain present. Long-term survivors (except those with successful transplants) often have residual abnormalities, such as thrombocytopenia, red cell macrocytosis, and elevated Hb F levels. Clearly we do not understand either the mechanism of action of this poorly characterized drug, the ultimate course of patients with ATG-induced remissions, or the basis of their partial recovery.
Cyclosporine.
Cyclosporine (formerly cyclosporin A) is a fungal cyclic undecapeptide and an effective immunosuppressive agent. Among its many effects, cyclosporine can inhibit T cells, preventing production of IL-2 and IFN-γ. About one half of patients who had failed ATG or ALG treatment subsequently experienced remission with cyclosporine. Treatment with cyclosporine alone yielded significantly lower response rates and higher rates of disease progression as compared with combined therapy.
Oral cyclosporine should be administered twice daily to maintain blood trough levels between 100 and 250 ng/mL as measured by radioimmunoassay, though the target levels may vary depending on laboratory methods used to determine cyclosporine levels. Hematologic responses can take weeks to months, and an initial trial period of 3 to 6 months is generally recommended.
Toxic effects from cyclosporine use are not insignificant and include hypertension, hypertensive encephalopathy, azotemia, hirsutism, gingival hypertrophy, coarsening of facial features, tremor, immunodeficiency, increase in serum creatinine levels, transient or irreversible nephrotoxicity, hepatotoxicity, seizures, and P. carinii pneumonia. As with ATG, in vitro tests of the effect of cyclosporine on progenitor cells do not predict responses.
Combination Immunosuppressive Therapy.
The combination of ATG with cyclosporine has resulted in higher rates of hematologic recovery than either drug alone. Importantly, intensive IST has greatly improved the results of therapy in two groups commonly refractory to ALG or ATG alone: children and patients with very severe disease. In the National Institutes of Health (NIH) Clinical Center trial, survival curves of those older and younger than 35 years of age were similar. A large study including both children and adults at the NIH reported a response rate of 67% at 43 months and a 5-year actuarial survival rate of 70% (86% for responders and 16% for nonresponders). The Gruppo Italiano Trapianti di Midolio Osseo (GITMO)/European Group for Blood and Marrow Transplantation (EBMT) study of 100 patients with severe aplastic anemia including children (median age 16 years) treated with ALG, cyclosporine, prednisolone, and G-CSF found trilineage hematopoietic response in 77% after one or more courses of ALG. Several trials in children have reported the efficacy of combined ATG and cyclosporine in the pediatric population with an overall response rate of close to 80% ( Table 6-2 ). Although greater lymphocyte depletion is achieved with rabbit ATG as compared to that with horse ATG, a randomized trial comparing horse ATG versus rabbit ATG in the upfront treatment of aplastic anemia demonstrated that superior hematologic response (68% versus 37%) and survival (96% versus 76%) was achieved with horse ATG.
