While surgical excision remains the gold standard for curative treatment of small renal cell carcinomas, ablative therapy has a place as a minimally invasive, kidney function–preserving therapy in carefully selected patients who are poor candidates for surgery. Although laparoscopic cryoablation and percutaneous radiofrequency ablation (RFA) are commonly performed, percutaneous cryoablation and laparoscopic RFA are reportedly being performed with increasing frequency. The renal function and complication profiles following ablative therapy are favorable, while oncologic outcomes lag behind those of surgery, thus reinforcing the need for careful patient selection.
The American Cancer Society estimates that more than 50,000 patients in the United States are diagnosed with renal malignancies each year and that 10,000 of these patients will die of their disease. Most renal malignancies are low-stage, low-grade renal cell carcinomas. Recently, diagnoses of small renal cell carcinomas have increased, especially among patients older than 70 years, in part because of the widespread use of abdominal ultrasonography and computed tomography (CT). Most renal cell carcinomas are detected incidentally.
Radical nephrectomy, once the primary treatment of small renal cell carcinoma, has been associated with a relatively high incidence of chronic kidney disease, cardiovascular mortality, and overall mortality, and is now considered overtreatment. Today, the standard of care for most patients with small renal cell carcinoma is surgical extirpation by partial nephrectomy, which provides disease control equivalent to that of radical nephrectomy but with the advantage of renal preservation However, some patients with small renal cell carcinomas, including elderly patients and patients with renal dysfunction are poor candidates for surgical extirpation. For these patients, radiofrequency ablation (RFA) or cryoablation may be a more appropriate treatment option.
Patients who qualify for ablative therapy should be considered to first have a biopsy of the renal mass for histologic confirmation of malignancy. Those confirmed to have a benign tumor may be counseled to only undergo surveillance. Patients need to be made aware of therapeutic alternatives, potential complications, need for strict imaging surveillance for an indefinite period of time, and the potential need for postablation biopsy to histologically confirm treatment success. Patients who are unable to meet these criteria should not be considered for ablative therapy.
Radiofrequency ablation
Mechanism of Action
In RFA, heat is generated by friction of water molecules reacting to radiofrequencies, and the heat destroys tumor tissue. Ex vivo and in vivo RFA of renal tumors was initially described by Zlotta and colleagues in 1997; since then, the use of RFA for treating renal masses has surged. Hsu and colleagues used light microscopy and a porcine model to study RFA-induced histologic changes in the renal parenchyma and found that chromatin blurring, increased cytoplasmic eosinophilia, loss of cell membrane integrity, and interstitial hemorrhage were among the earliest changes following RFA. Three days after RFA, Hsu and colleagues noted extensive coagulative necrosis with early infiltration of fibroblasts and acute inflammatory elements at the border of the RFA. At 1 week, nuclear degeneration was more pronounced, and acute inflammatory elements had increased. At 2 weeks, nuclear degeneration was complete; and at 4 weeks, loss of cytoplasmic borders and features characteristic of renal parenchyma were present. Renal parenchyma 1 cm from the ablation zone appeared histologically normal. At 3 months, near-total resorption of the lesion had occurred.
Cell death resulting from RFA begins after 5 minutes of exposure to temperatures at or more than 50°C. However, temperatures greater than 105°C are counterproductive because they cause acute tissue vaporization and eschar formation that limits heat conduction and thus the efficacy of RFA. For effective thermal ablation, homogeneous RFA electrode temperatures in the range of 50°C to 100°C must be maintained throughout the target area. Multitined probes, saline-cooled probes, temperature or impedance monitoring, and other mechanisms are used by various manufacturers to maintain ideal temperatures.
Technique
At our institution, we typically perform a core needle biopsy of the renal mass using a 20-gauge coaxial needle to confirm malignant histology before performing RFA. After renal cell carcinoma is confirmed by permanent section analysis, RFA can then be performed percutaneously under CT guidance or laparoscopically under direct visualization and intraoperative ultrasonography guidance.
The percutaneous RFA technique that we use has been described in detail previously but is briefly reviewed here. Percutaneous RFA is performed with the patient under general anesthesia. We and others believe that general anesthesia is key to localizing and targeting small renal masses accurately; other investigators prefer using intravenous sedation. The patient is placed in the prone position, and CT is used to localize the tumor. Ablation is performed using a 200-W impedance-based device. One or more electrodes are positioned in the tumor under CT guidance. Then, sequential overlapping ablations are performed as indicated by the size and location of the tumor until the tumor is completely ablated.
In patients in whom the renal lesion and colon are in proximity to one another, hydrodissection is performed by injecting saline in a plane between the kidney and colon to improve the safety of RFA. Similarly, cooled saline can be injected in a retrograde fashion through the ureter to protect it from heat damage. Additional maneuvers to safely target the lesion include torquing the RFA probe manually and positioning an angioplasty balloon between the tumor and bowel.
Immediately after RFA, intravenous contrast material can be administered to assess the ablation zone and adequate ablation of the lesion.
Lesions that cannot be safely treated by percutaneous RFA, such as anterior tumors and tumors close to the renal hilum or ureter, can be treated with transperitoneal laparoscopic RFA. To avoid damage to the renal hilum, ureter, or adjacent normal structures, transperitoneal laparoscopic RFA is performed with the patient under general anesthesia. The patient is placed in the modified flank position. A Veress needle is used to access the peritoneum, 3 laparoscopic ports are placed, the colon is mobilized medially, the kidney is identified, and the ureter mobilized away from the treatment area if necessary. Perinephric fat (except for the fat in immediate contact with the tumor) is mobilized away from the kidney. Intraoperative ultrasonography is then used to assess the extent of the tumor and the tumor’s relation to adjacent structures.
Before ablation is performed, small cautery marks that will serve as visual landmarks to aid in repositioning the probe are made at the margin of the proposed treatment zone. This is done because as treatment proceeds, the lesion contracts and anatomic landmarks become subtle or disappear on ultrasonography. The RFA probe is then inserted into the tumor under ultrasound guidance until the probe tip is at or just past the deepest tumor-renal parenchyma interface. Optimal initial probe targeting is key; as RFA starts, tissue vaporization and cavitation degrade the ultrasound image, diminishing accurate targeting capability. Treating the deepest margin first effectively achieves a vascular amputation such that subsequent, more superficial overlapping ablations can be completed more quickly. Ablation is performed using a 200-W impedance-controlled device following a standardized protocol. Each initial session is followed by a second confirmatory session, after which the ablation probe is partially withdrawn and another sequential ablation performed. The probe is repositioned as necessary, and the process is repeated until the entire tumor and margin are treated. The perirenal fat is then approximated with absorbable sutures, and a standard laparoscopic exit is performed.
Cryoablation
Mechanism of Action
Four thermal parameters are used to determine the rate of cellular damage during cryoablation: cooling rate, minimum temperature reached, time maintained at the minimum temperature, and thawing rate. Cryoablation directly kills tumor cells by causing osmotic dehydration that damages enzymatic pathways, organelles, and the cell membrane and by causing intracellular ice formation that supercools the cytoplasmic contents. Cryoablation also indirectly kills tumor cells by targeting small blood vessels, which results in a hypoxic microenvironment in which tumor cells cannot survive. To ensure adequate tumor treatment, a temperature of −40°C must be achieved throughout the tumor; this typically occurs when the ice ball extends 0.5 to 1 cm beyond the tumor margins.
The immunologic sequelae after cryoablation are interesting and potentially of clinical relevance. Recently, we investigated the immunologic sequelae of cryoablation in a murine model of renal cell carcinoma and found increased lymphocytic infiltration in cryoablated lesions, especially in areas surrounding blood vessels and areas of sublethal tissue injury. Neutrophils, macrophages, and CD4+ and CD8+ T cells with an increased ratio of T helper type 1 cells to T helper type 2 cells were the most common immune reaction components. In addition, cryoablated lesions showed increased interferon-gamma production. There is great interest in developing combinatorial strategies using cryoablation and immunotherapeutic modulation for treatment of metastatic renal cell carcinoma.
Technique
Depending on the technology available, experience of the attending surgeon, and location and size of the tumor, cryoablation can be performed using an open, percutaneous, or laparoscopic approach. The laparoscopic approach is similar to that used for RFA, with the notable exception that ultrasound can and should be used throughout the freezing process to monitor the ice ball. Percutaneous cryoablation is performed similar to RFA as previously described. Cryoablation units use argon gas to achieve rapid freezing at the tip of the probe. The benefit of argon gas is that smaller-diameter probes can be used, and the ice ball formation terminates at the end of the freezing cycle. As a result of the Joule-Thomson effect, depressurized argon gas has temperatures ranging from −80°C to −195°C. Helium, which follows the reverse effect to release heat as it is depressurized, is thus used for the thawing cycle. Typically, 2 freeze/thaw cycles are performed, and multiple probes can be placed in the tumor if one probe is not sufficient to cover the entire tumor and its margin. The ice ball is usually monitored using magnetic resonance imaging or CT fluoroscopy during percutaneous procedures and with a steerable laparoscopic ultrasonography probe during laparoscopic procedures.
Follow-up and outcomes after ablative therapy
Routine follow-up after RFA includes medical history, physical examination, chest imaging with conventional radiography or CT, complete metabolic profiles, and other diagnostic tests for potential metastatic locations. We typically obtain contrast-enhanced CT or magnetic resonance images of the RFA lesion within 6 weeks of initial therapy, and then at 6, 12, 18, and 24 months. Depending on the imaging study results, semiannual or annual follow-up is performed thereafter.
Definition of Local Treatment Success
Because the tumor is not excised after ablation and thus no tissue is available for histopathologic analysis, radiographic studies must be used to determine treatment success. Successful ablation is typically defined as a lack of enhancement after injection of intravenous contrast material and tumor involution. However, unlike lesions treated with cryoablation, RFA-treated lesions do not always involute, complicating the assessment of success, and reducing it to just absence of enhancement. Matsumoto and colleagues studied the natural radiological history of 64 RFA-treated renal tumors and found that endophytic tumors developed a low-density, nonenhancing, wedge-shaped defect with fat infiltration between the ablated tissue and normal renal parenchyma, but that exophytic tumors retained a configuration similar to that of the original tumor with a lack of contrast enhancement and minimal involution. However, in cases in which no enhancement and minimal involution were present, we found that biopsy of the ablation zones 6 months after RFA revealed viable tumor cells in about 10% of cases (data presented at Genitourinary Cancers Symposium, American Society of Clinical Oncology Annual Meeting 2010, San Francisco). Others have also reported finding viable cancer cells in the absence of contrast enhancement. There can be not only false-negative imaging findings but also false-positive imaging findings can occur and alter clinical management. Therefore, if ablation zones increase in size or fail to involute even in the absence of contrast enhancement 6 months after the initial ablation, we routinely obtain multiquadrant core biopsy specimens. The potential need for biopsy after ablation should be discussed with the patient, who must accept it as part of the treatment plan even before ablative therapy is initiated.
Functional Outcomes
Ablative therapy seems to cause less renal function loss than partial nephrectomy and certainly much less than radical nephrectomy. Lucas and colleagues used the Modification of Diet in Renal Disease equation to compare renal function in patients who underwent RFA, partial nephrectomy, or radical nephrectomy and found that the rate of 3-year freedom from stage 3 chronic kidney disease (a decrease in glomerular filtration rate [GFR] of <60 mL/min/1.73 m 2 ) was 95.2% in patients who underwent RFA, 70.7% in patients who underwent partial nephrectomy, and 39.9% in patients who underwent radical nephrectomy ( P <.001).
In a multi-institutional study, Raman and colleagues evaluated renal function changes in patients with solitary kidneys and found that patients who underwent open partial nephrectomy under cold ischemic conditions had a greater decrease in GFR than patients who underwent RFA at all evaluated times, including soon after the procedure (15.8% vs 7.1%; P <.001), 12 months after the procedure (24.5% vs 10.4%; P <.001), and at last follow-up (28.6% vs 11.4%; P <.001). Weisbrod and colleagues recently published the results of a large cohort of patients with solitary kidneys treated by percutaneous cryoablation, showing no patients needing dialysis and minimal change in GFR.
Differences Between Radiofrequency Ablation and Cryoablation
There are 2 key differences between cryoablation and RFA. First, in laparoscopic cryoablation, ultrasonography is eminently useful for monitoring the cryolesion; however, in laparoscopic RFA, ultrasonography cannot be used to effectively monitor the lesion because image quality starts to degrade as soon as treatment is initiated. Second, because multiple sequential treatments, which can be performed during RFA, cannot be performed during cryoablation, the initial geometric targeting by probe placement is critical in cryoablation. Biologic differences between the 2 modalities also probably exist, as RFA causes immediate vascular coagulation and thrombosis, whereas after cryoablation there is a period of vascular flow, vascular congestion, subsequently followed by thrombosis. This period of reestablished vasculature may provide greater exposure of tumor-associated antigens to immune cells.
Oncological Outcomes
The results of recent RFA and cryoablation series are summarized in Tables 1 and 2 . Differences in reporting criteria and quality among studies should be noted when reviewing the ablation literature but can be difficult to discern. Definitions of incomplete ablation vary. The number of RFA sessions can vary, and success can be reported after the first, second, or third separate session. Some investigators report overall success regardless of the number of ablation sessions required. Because treatment success can be reported by measuring the rate of incomplete ablation, local recurrence at the site of ablation, recurrence in the contralateral kidney, or bona fide metastasis, success must be clearly defined when reporting the findings of these studies. In addition, biopsies are not routinely performed before treatment and are performed even less frequently after treatment; the fact that about 25% of small renal masses are benign could confound reporting of recurrence or survival outcomes because a substantial number of patients may have had benign lesions to begin with. When biopsy results are available, outcomes should be stratified according to pathology findings. In addition, most studies report on success in terms of simple percentages without accounting for the fact that different patients are followed for different periods; the appropriate method would be to use a Kaplan-Meier plot, which accounts for patient censoring. Adding to the difficulties in evaluating the data is that the growth rate of small renal tumors may be very slow ; thus, in most patients the follow-up period is too short to draw definitive conclusions. These data deficiencies and limitations, which can also be attributed to much of the published literature on open surgery, were highlighted in the recent publication of the American Urological Associations Guidelines for Treatment of the Renal Mass.
Author, Year | No. of Patients | No. of Tumors | Mean Tumor Size, cm (Range) | Method | Mean Follow-up Time, Months (Range) | Local Recurrence/Incomplete Ablation |
---|---|---|---|---|---|---|
Ahrar et al, 2005 | 29 | 30 | 3.5 (SD, 0.2) | P | 10 (1–33), median 7 | 1/24 |
Mahnken et al, 2005 | 14 | 15 | 3 (SD, 1) | P | 13.9 (SD 12.4) | 0/15 |
Gervais et al, 2005 | 85 | 100 | 3.2 (1.1–8.9) | P | 27 (3–72) | 9/85 |
Matsumoto et al, 2005 | 91 | 109 | 2.4 (0.8–4.7) | P, L, O | 19 (12–33) | 3/91 |
Arzola et al, 2006 | 23 | 27 | 2.7 (0.9–6) | P | 24 (7–53) | 4/20 |
Park et al, 2006 | 78 | 94 | 2.4 (1–4.2) | P, L | 25 (12–48) | 5/94 |
Carey et al, 2007 | 36 | 37 | NR (3–5) | P, L | 11.3 (1–44) | 3/37 |
Stern et al, 2007 | 40 | 40 | 2.4 (NR) | P, L | 30 (18–42) | 3/40 |
Breen et al, 2007 | 97 | 105 | 3.2 (1.1–6.8) | P | 18 (1–76) | 22/105 |
Zagoria et al, 2007 | 104 | 125 | 2.7 (0.6–8.8) | P | 13.8 (1–76), median 9.8 | 16/125 |
Lucas et al, 2008 | 86 | 86 | 2.3 (2.2–2.5) | P, L | Median 40 (NR) | 6/86 |
Levinson et al, 2008 | 31 | 31 | 2.1 (1–4) | P | 61.6 (41–80), median 62 | 4/31 |
Wingo et al, 2008 | 39 | 41 | 2.7 (1–5) | P, L | 29 (NR) | 4/41 |
Gupta et al, 2009 | 151 | 163 | 2.3 (1–5.4) | P | 18 (1.5–70) | 5/163 |
Hoffmann et al, 2010 | 10 | 13 | 2.7 (1.9–4.2) | P | NR (3–24) | 0/13 |
Raman et al, 2010 | 47 | 53 | 2.7 (1.5–6.5) | P, L | Median 18 (6–66) | 5/47 |
Del Cura et al, 2010 | 58 | 65 | 3.1 (1.2–5.3) | P | 26.5 (10–50) | 12/58 |
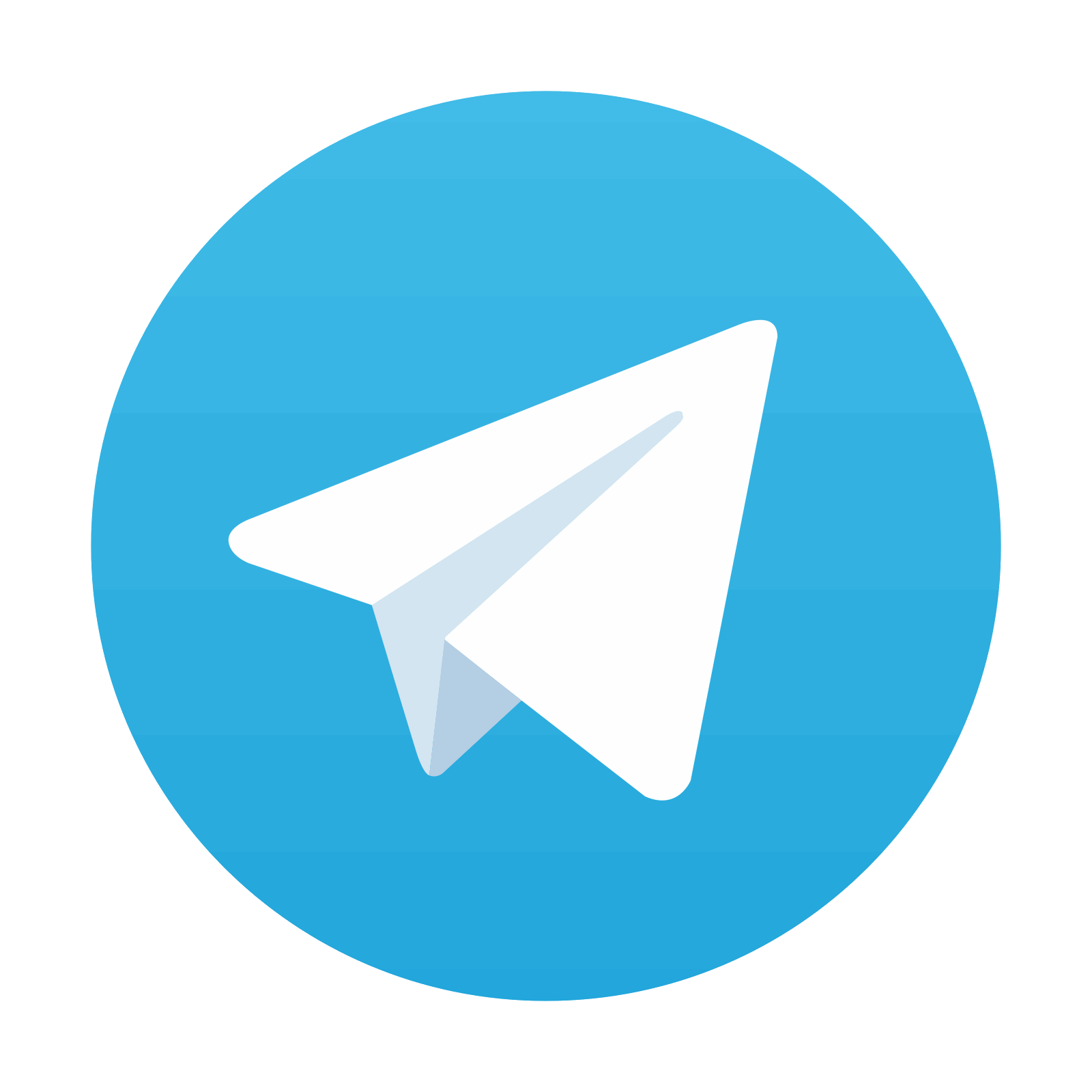
Stay updated, free articles. Join our Telegram channel

Full access? Get Clinical Tree
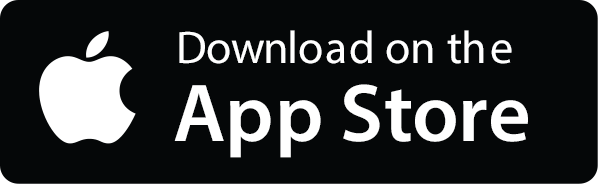
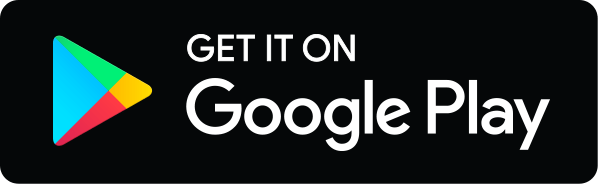
