Highlights
- •
Prostate cancer is associated with the genetic components.
- •
The understanding of the genetics components of prostate cancer will help researchers in developing mitigating approach.
- •
GWAS provides a detailed method for identifying the distinct sequence of a gene that is associated with cancer risk.
Abstract
Several studies indicated that prostate cancer has a hereditary component. In particular, a significant risk of prostate cancer has been linked to a tight familial lineage. However, to provide insight into how prostate cancer is inherited, characterising its genetic profile is essential. The current body of research on the analysis of genetic mutations in prostate cancer was reviewed to achieve this. This paper reports on the effects and underlying processes of prostate cancer that have been linked to decreased male fertility. Many research approaches used have resulted in the discovery of unique inheritance patterns and manifest traits, the onset and spread of prostate cancer have also been linked to many genes. Studies have specifically examined Androgen Receptor gene variants about prostate cancer risk and disease progression. Research has shown that genetic and environmental variables are important contributors to prostate cancer, even if the true origins of the disease are not fully recognised or established. Researchers studying the genetics of prostate cancer are using genome-wide association studies more and more because of their outstanding effectiveness in revealing susceptibility loci for prostate cancer. Genome-Wide Association Studies provides a detailed method for identifying the distinct sequence of a gene that is associated with cancer risk. Surgical procedures and radiation treatments are 2 of the treatment options for prostate cancer. Notwithstanding the compelling evidence shown in this work, suggests that more research must be done to detect the gene alterations and the use of genetic variants in the treatment of prostate cancer.
Graphical Abstract
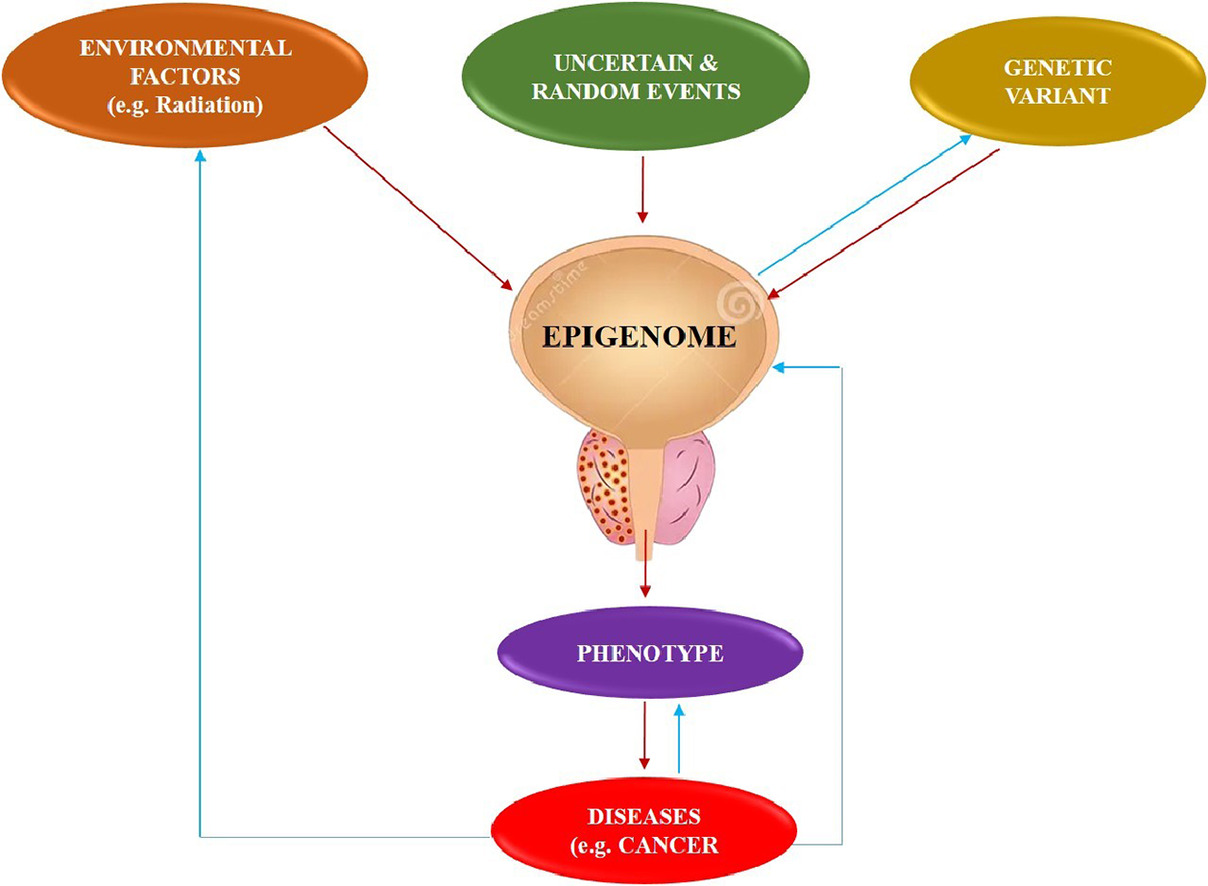
1
Introduction
The prostate gland is an integral component of the male reproductive system, situated inferior to the bladder and anterior to the rectum in men [ ]. The prostate gland, measuring about the size of a walnut, is responsible for the production of seminal fluid and facilitates the movement of sperm [ ]. Prostate cancer ranked as the third most prevalent form of cancer worldwide, primarily affects this gland [ ]. In 2020, the World Health Organization reported that prostate cancer was detected in more than half of the nations in the world (112 out of 185). The incidence of this disease exhibits variations across countries categorized by their Human Development Index (HDI), distinguishing those with high HDI from those with low HDI [ ].
Prostate cancer mostly impacts the elderly male population, particularly those aged 70 to 74 years. Consequently, the occurrence and fatality rates of this disease exhibit a significant correlation with advancing age [ ]. Also, around 10% to 15% of males diagnosed with prostate cancer have a minimum of 1 affected relative [ ]. According to Rawla [ ], there is a notable disparity in the incidence rates and aggressiveness of prostate cancer between African-American males and white men. Due to the high heritability of prostate cancer, a familial predisposition may elevate the likelihood of developing the disease [ ]. However, considerable evidence suggests that genetics probably exerts a significant influence, at least among males who have a favorable familial lineage [ ].
Numerous studies have established a potential correlation between diet and prostate cancer, as well as obesity [ , , ]. Specifically, an elevated risk of developing prostate cancer has been associated with calcium-rich diets [ ]. As the prostate cancer organ gradually enlarges, symptoms manifest, which frequently impact the urinary and sexual functions of males [ , ]. Frequent and excruciating urination [ ], or even incapacity to urinate [ ], may result from an enlarged prostate compressing the urethra and obstructing urine passage [ ]. The severity of the illness and the time of diagnosis determine the course of treatment. Millions of men have small clusters of ostensibly benign early-stage prostate cancer.
It is common for physicians to regularly check prostate-specific antigen (PSA) levels and do physical exams in order to track the disease’s development [ ]. If the illness progresses slowly, it may often be controlled in this manner for years. If the patient is well enough to undergo the procedure and the tumour is confined, surgery can be an additional course of therapy; prostate cancer may potentially be treated with chemotherapy [ ]. Chemotherapy and androgen-targeted therapy are now the major forms of treatment used for men with metastatic castration-resistant prostate cancer (mCRPC) [ ].
2
Search strategy: Defining the genetic profile of prostate cancer
The current analysis makes use of published data from academic, peer-reviewed publications. Articles were evaluated using Medical Subjects Heading (MeSH) indexes and pertinent keywords from African Journals Online (AJOL), Directory of Open Access Journals (DOAJ), Google Scholar, PubMed/PubMed Central, and Scopus. The keyword format used was to generate a broad search on Prostate Cancer or Prostate Carcinoma and to pair this search with another keyword on Genetics or Genetic Profile. The keywords and syntax generated was (Prostate Cancer) OR (Prostate Carcinoma) AND (Genetics) OR (Genetic Profile). The search period ranged from establishment of database till 2023. Only articles published in English language were included in this review.
2.1
Genetic profile of prostate cancer
Several studies have shown hereditary factor in the development of prostate cancer [ , , , ]. Notably, some studies have shown a correlation between a strong family lineage and an elevated susceptibility to prostate cancer [ ]. Research has shown the presence of genetic alterations in hereditary prostate cancer, which increase the predisposition of some individuals to develop prostate cancer. In their research, Sekhoacha et al. [ ] examined the need for unique prostate cancer screening regimens for African American males. The study found notable variations in prostate cancer incidence and outcomes across different ethnic and racial groups. The basis for this discovery is rooted in the observation that individuals of African heritage exhibit the greatest rates of occurrence and death, which might be attributed to genetic factors [ ]. Furthermore, there is compelling evidence linking genetics to the vulnerability of developing prostate cancer. This evidence encompasses various approaches, such as family-based genetic linkage analysis, genome-wide association studies, and the presence of inherited mutations in well-established cancer predisposition genes among individuals with a family history of other cancers. In general, the classification of the genetic makeup of prostate cancer has been essential for determining different forms of the illness and the corresponding treatment approaches [ ].
2.2
Common genetic mutations and variants associated with prostate cancer
Various studies have used a range of research methodologies to investigate the genetic variability associated with prostate cancer. The use of diverse research methodologies has resulted in the recognition of various patterns of inheritance and observable traits [ ]. Linkage studies are performed on high-risk families to identify genes or genetic polymorphisms that make individuals more vulnerable to prostate cancer. Statistical evidence was used to determine if a genomic locus has a gene that makes an individual susceptible to a disease. A noteworthy gene that has been found in linkage analysis as a susceptibility gene is HOXB13 [ ]. Pathogenic mutations in this gene are often detected in families with several affected individuals or as evidence of illness starting at an early age. A multinational investigation of 2443 prostate cancer families identified 112 families of European heritage that have the HOXB13 pathogenic mutation. According to Xu et al. [ ], the research found that the carrier rate among afflicted males in the target households was substantially higher at 47% compared to unaffected men at 31%.
Another approach is case-control research, which aims to discover a particular genetic variation by comparing occurrences and controls based on many criteria. Specifically, research has examined the genetic variation in the Androgen Receptor (AR) gene regarding the progression of diseases and the likelihood of developing prostate cancer [ ]. The emphasis on AR is linked to its presence in all stages of prostate carcinogenesis. Furthermore, the elimination of an AR signal may lead to the regression of prostate cancer [ ]. Admixture mapping is a research tool used to identify genetic variations associated with disease features in individuals of mixed ancestry [ ]. Population-specific genetic variables are found based on differences in illness incidence among 2 or more ancestral populations [ ]. This approach is often used for identifying genetic loci associated with elevated prostate cancer risks in individuals of African American descent. The higher incidence of prostate cancer among African American males estimated to be 64% higher compared to those of European ancestry suggests that there are specific genetic variations that contribute to this disparity [ ]. These genetic variations are expected to be found in areas of the genome that have a higher proportion of African ancestry [ ].
2.3
Genes implicated in the development and progression of prostate cancer
Several genes have been associated with the development and advancement of prostate cancer. Specifically, research has examined the genetic variation of the AR gene in relation to the progression of diseases and the likelihood of developing prostate cancer [ ]. The emphasis on AR, a protein that is stimulated by androgens such as testosterone, is closely related to its involvement in all stages of prostate cancer development. Hence, genetic polymorphisms in the AR gene may be associated with an increased susceptibility to prostate cancer as well as a reduced responsiveness to hormone treatment. BRCA1 and BRCA2 genes have been identified as playing a role in the development and progression of prostate cancer [ , ]. These genes are mostly associated with an elevated susceptibility to ovarian and breast cancer in females. However, mutations in these genes that repress tumor growth, especially BRCA2, might lead to an augmented likelihood of developing prostate cancer in males. Furthermore, HOXB13 plays a significant role in the formation of the prostate gland. Therefore, genetic alterations in this specific gene may lead to an increased susceptibility to prostate cancer at a younger age, particularly among individuals of European descent [ ]. Table 1 shows 10 genes that are clear cut genes for PSA and the p value for the possibility that both gene occur.
S/N | Gene changes associated with prostate cancer in Africa | References |
---|---|---|
1. | Forehead box A1 (FOXA1), through direct interactions with the AR, helps to shape AR signaling driving the growth and survival of normal prostate and prostate cancer cells and also possesses an AR-independent role to regulate the epithelial-to-mesenchymal transition (EMT). Increase mutations of FOXA1 in African Men define a unique subset of prostate cancers | [ , , , , ]. |
2. | Tumor protein (TP53) gene instructs cells to make tumor protein 53, that helps detects damaged DNA and determining if such DNA can be fixed or cause to self-destruct to prevent replication and potential cancer causing DNA. The mutation of TP53 leads to Cancer | [ , , , ] |
3. | AR gene encodes a nuclear receptor activated following the binding of androgenic hormones to regulates the transcription of several growth and differentiation-related genes required for the survival of prostatic cells. Mutations in the AR gene cause a range of phenotypic abnormalities of male sexual development | [ , , , , ] |
4. | BRAF, a key signaling activator of the mitogen-activated protein kinase (MAPK) pathway coordinated by the RAS proteins. The BRAF mutations are additional drivers of prostate cancer development in Africa men | [ , , ]. |
5. | Germline gene mutation including the BRCA2 and BRCA1 mutations. men with BRCA2 mutations present at a younger age with higher Gleason grade tumors, higher rates of nodal involvement and distant metastases at diagnosis, are at higher risk of prostate cancer. BRCA1, ATM, CHEK2, and PALB2 are also involved in homologous recombination DNA repair and have been associated with increased prostate cancer risk | [ , , , , , , ]. |
6. | RNASEL, a candidate hereditary prostate cancer gene (HPC1) located at chromosome 1q25, encodes ribonuclease L (RNASEL), an enzyme in the interferon (IFN)-induced antiviral 2–5A system. It employs several mechanisms to fight viral infections, including the cleavage of viral RNA and induction of apoptosis. The mutations in the gene may reduce the ability to fight viral infections and hence the progression of prostate cancer | [ , ]. |
7. | ELAC2 located at chromosome 17p12 is a ubiquitously expressed enzyme potentially involved in tRNA processing and cell signaling pathways. It is of particular interest in prostate cancer as the sequence variants of the gene have been suggested to play a role in genetic susceptibility to hereditary and sporadic forms of the prostate cancer thus, the mutations of the ELAC2 gene have been found to confer increased prostate cancer susceptibility in families. | [ , , ]. |
8. | The MSR1 gene at 8p22 has been suggested as a candidate gene for hereditary prostate cancer as the germline variants have been reported to be associated with the prostate cancer development. | [ , , , ] |
9. | The homeobox B13 (HOXB13) gene is expressed in the prostate beginning in early development affecting the prostate cell proliferation and differentiation with the regulation of the androgen receptor. The (HOXB13) G84E mutation are used in genetic counselling for prostate cancer due to it heterogeneity. | [ , , , , ] |
2.4
Prostate cancer and genetic inheritance
While the exact causes of prostate cancer remain partially unknown, scientific investigation has shown that genetic and environmental variables significantly contribute to the development of this disease [ ]. Hence, several investigations have shown a substantial correlation between prostate cancer and genetic heredity. It may be inferred that certain genetic variables are associated with the likelihood of developing prostate cancer [ ].
Lange [ ] found that males who have a familial predisposition to prostate cancer are at a heightened risk of getting the condition. This suggests that there could be certain genetic variables that are passed down from parents that greatly raise the likelihood of developing prostate cancer. Therefore, those who have a father or brother with prostate cancer are at a higher risk of having the condition. Moreover, Li et al. [ ] said that some hereditary gene mutations, such as BRCA1 and BRCA2, might increase the likelihood of getting aggressive prostate cancer at a younger age.
2.5
Genetic profiling techniques applicable to prostate cancer and their advantages
Prostate cancer has a diverse genetic profile, unlike other types of tumours [ ]. This confirms the presence of many genetic profiling methods that may be used for prostate cancer. One of the most important approaches is next-generation sequencing, a powerful sequencing technology that can simultaneously sequence millions of DNA fragments in a high-throughput manner [ ]. This allows for the detection of genetic mutations and changes in genes related to prostate cancer. This may aid in the diagnosis, selection of therapy, and monitoring of disease progression. According to the 2017 experiment by Kamps et al., this method is noteworthy for its exceptional sensitivity and capacity to detect a wide range of genetic alterations, both in the germline and somatic cells.
Moreover, polymerase chain reaction is a crucial method that allows for the amplification, detection, and analysis of DNA sequences [ ]. This technology facilitates the detection of mutations in certain genes associated with prostate cancer, and it is particularly noteworthy for its robust capacity to detect minimal levels of DNA and analyse tiny quantities of genetic material. Another method is fluorescence in situ hybridization, which is a cytogenetic technique that employs fluorescent probes to identify specific DNA sequences on chromosomes. The use of this method allows for the identification of gene amplification, deletions, and translocations in prostate cancer cells. Its notable advantage is its capacity to accurately detect specific genetic changes and conduct a thorough examination of individual cells [ ].
2.6
Epigenetics and cancer
A significant obstacle in cancer therapy is the occurrence of cancer metastasis, which requires a thorough comprehension of how cancer cells develop and sustain their metastatic traits [ ]. Studies have shown that certain reversible epigenetic processes regulate the first stages of metastasis. Hence, epigenetics has been recognized as playing a significant role in the diagnosis, treatment, and prevention of cancer [ ].
Epigenetic alterations refer to the addition or removal of chemical groups from the DNA [ ]. A prominent change is DNA methylation, which involves the addition of a methyl group to cytosine residues in DNA [ ]. While promoting changes in enzyme expression that regulate epigenetic modifications, epigenetic abnormalities can occur. Some of the most important epigenetic changes in prostate cancer are DNA methylation, histone modifications, noncoding RNAs, and chromatin remodelling. Hence, a comprehension of the epigenetic modifications in prostate cancer might potentially lead to the development of novel diagnostic and therapeutic approaches. For example, DNA methylation patterns may be used as biomarkers to diagnose and predict the outcome of cancer. In addition, medications that specifically target epigenetic alterations may potentially be developed as promising therapeutics for prostate cancer [ ].
3
Overview of Genome-Wide Association Studies (GWAS) in prostate cancer
Genome-wide association studies (GWAS) provide a comprehensive method for identifying specific genetic variations, known as genetic variants that are linked to the risk of developing cancer. This approach is not limited by existing knowledge and has the potential to detect genetic variants that are associated with a lower risk of cancer which also have a significant impact on public health [ ]. According to Juran and Lazardis [ ], GWAS has become a widely used tool for researchers studying the genetic causes of prostate cancer. It is very effective in identifying specific regions of the genome that are associated with an increased risk of developing prostate cancer.
Genome-wide linkage analysis is a powerful method used before GWAS to identify the genetic basis of a disease. However, the combination of several genetic and environmental variables plays a crucial role in defining the majority of illnesses. The study of linkage, also known as linkage studies, is less effective in detecting genetic variations associated with certain disorders [ ].
The process of conducting GWAS often occurs in many phases. Large cohorts of patients and controls undergo DNA genotyping using commercially accessible microarray chips. Despite the recent decrease in genotyping costs, the expense of genotyping a diverse population with numerous single nucleotide polymorphisms (SNPs) remains substantial. According to Elston et al. [ ], multistage designs may retain a significant portion of the design’s power while being just half as expensive. In general, investigations often have 2 or 3 parts. Initially, SNPs across the whole genome are contrasted between patients and controls to identify those that could be linked to the risk of developing cancer. Subsequently, only the top 5% to 10% of the most significant SNPs advance to the subsequent phase, which entails an even bigger group of unrelated patients and controls. Finally, these SNPs are examined in larger and more varied groups of patients and controls [ ]. To achieve effective GWAS, it is crucial to emphasise the importance of repetition. The key factor in the GWAS is in the process of replication that is repeated to ensure the credibility of the result obtained [ ]. Fig. 1 below shows the steps in the analysis of genome-wide linkage during the identification of genetic background of diseases
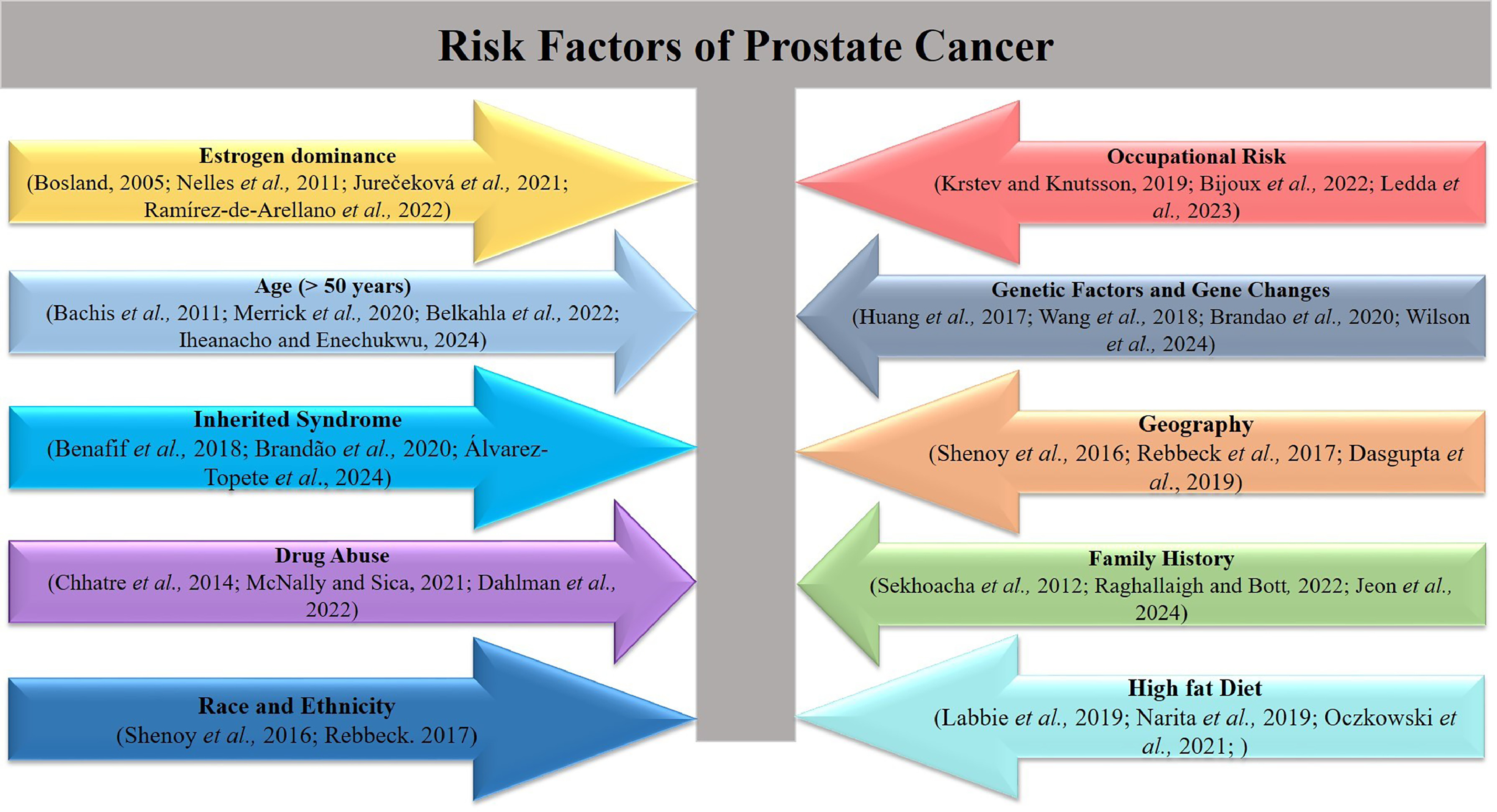
4
GWAS findings in prostate cancer
Through GWAS, enough genetic variations can be found to give a genetic diagnostic score and a risk prediction score, which makes it easier to stay healthy. Nevertheless, a retrospective analysis conducted on the Chinese population revealed that these specific SNPs had little efficacy in diagnosing prostate cancer [ ]. The area under the receiver operating characteristic curve of the receiver operating characteristic was 0.604, which was inferior to that of the PSA. The introduction of SNPs in the European population may be the underlying factor contributing to this subpar performance. However, the findings of the REDUCE experiment [ ] indicate that prostate cancer prognosis is not solely dependent on this feature. Incorporating a genetic score in addition to recognised clinical characteristics might lead to a little improvement in Principal Component Analysis (PCa) prediction. An assessment of genetic markers, namely 33 SNPs, might be used to determine the need for a repeat biopsy. The absence of unexplored variations may account for this outcome and indicate the need to include more criteria for the prediction of prostate cancer risk. In addition, the efficacy of these SNPs in predicting prostate cancer prognosis during active monitoring of the cohort was evaluated, although the findings were suboptimal [ ].
5
Implication of GWAS findings for prostate cancer risk prediction and personalized treatment
GWAS findings are often deemed impractical in a clinical context owing to the modest impact of genetic variations on illness risk and their restricted contribution to prevalent diseases. This statement may be accurate since a finding that is “significantly associated” does not necessarily imply practical significance. For instance, research discovered a single nucleotide polymorphism (SNP) that influences height, although the effect size was just 0.44 cm [ ]. Studies have shown that the majority of loci associated with diseases have a small impact size [ ]. Under such circumstances, it is challenging to definitively determine the relevance of the gene to prostate cancer. Furthermore, it is important to consider that certain low-odds ratios observed in GWAS may not be solely attributed to the study itself. To draw accurate conclusions, it is necessary to examine the study’s particulars. For instance, employing pooled control groups from the general population as controls may diminish odds ratios due to the potential inclusion of individuals who could have been participants in the control group.
Genetic testing presents a significant obstacle in determining the pathogenicity of Variants of Uncertain Significance since there is not enough evidence to determine whether they are harmless or disease-causing. Therefore, it is recommended to exclude variants of uncertain significance from consideration since they cannot be used for clinical diagnosis or choices on treatment plan [ ].
6
Overview of next-generation sequencing and application in prostate cancer research
Next-generation sequencing (NGS) is a contemporary method for studying infections. The technology employs a very efficient sequencing apparatus capable of rapidly analysing millions of minute DNA fragments over several hours. The length of each fragment ranges from 150 to 500 bases, depending on the specific NGS platform used. Most of the sequenced fragments are from human cells, with just a small proportion (0.1%–8%) obtained from the pathogenic bacterium [ ]. The sensitivity and sequencing capacity of DNA have been significantly enhanced by NGS [ ]. The NGS comprises many methodologies, including whole genome sequencing, whole-exome sequencing, RNA sequencing, reduced representation bisulfite sequencing, and chromatin immunoprecipitation sequencing [ ].
Despite significant progress in the genetic profiling of prostate cancer, there is still a paucity of differentiation in aggressiveness and the identification of tumours with actionable therapeutic targets. Furthermore, the causative factors of the condition remain unidentified. The unique diversity of prostate tumours poses challenges in the tumour’s early detection of carcinogenic alterations, which play a crucial role in the development of a tumour’s evolutionary lineage [ ]. Nevertheless, there have been recent advancements that might potentially surmount these obstacles. Noninvasively collected materials, such as circulating tumour cells or exosomes found in blood or urine, may be analysed using NGS-based technologies. This analysis can provide valuable information on drug resistance and suggest alternative therapies that may improve patient lives. There is a growing interest in third-generation sequencing technology, which can sequence individual DNA and RNA molecules. This technology requires less tissue and eliminates the need for PCR amplification, resulting in a cost reduction [ ]. It enable multiple gene targets between nanograms of DNA with the cost, projected test volume, intended genomic target breadth, and required sensitivity considered when deciding which NGS platform to use [ ] and is particularly beneficial in primary settings where tumour specimens are limited due to formalin-fixed paraffin-embedded biopsies [ , ]. An amalgamation of imaging, pathology, and genomes has been proposed as a potent instrument in the identification, prediction, and treatment of medical conditions. The process of fragmenting genomic DNA and subjecting it to specifically designed oligonucleotides allow for the collection of exonic DNA, which corresponds to the functional regions of the genome. The DNA that has been enhanced is then amplified and sequenced using the methods outlined in Cosart [ ]. Genome analysis is efficiently conducted at a reasonable cost, and typically, coverage of 100 to 200 times accurately detects somatic mutations with high confidence. Increased coverage and the use of high-fidelity polymerases help to mitigate any minor errors that may occur during polymerase chain reaction amplification. Additionally, selective sequencing of only 2% of the genome can also introduce errors. Therefore, whole exome sequencing is currently the most widely used method in clinical diagnostics [ ].
Advancements in NGS technology have significantly enhanced our understanding of pyruvate carboxylase biochemistry and clinical variability. The pathways involved in the development of prostate tumours, including AR-signaling, phosphatidylinositol 3-kinase- Phosphatase and tensin homolog-protein kinase B (PI3K-PTEN-Akt), and Receptor Tyrosine Kinase (RTK)/Ras GTPase/MAP kinase (MAPK) (RTK-Ras-MAPK) pathways, have been elucidated by DNA-Seq, RNA-Seq, chromatin immunoprecipitation-Seq, and methyl-Seq investigations [ ]. Two investigations demonstrated the feasibility of doing extensive patient screening for pyruvate carboxylase during routine diagnosis [ ]. Analysing cancer cells extracted from transrectal ultrasonography needle-core biopsy specimens is possible [ ].
7
Next-generation sequencing technology application in diagnosis, prognosis, and treatment of prostate cancer
The novel sequencing technique has remarkable attributes, allowing for the execution of the sequencing process in a highly parallel manner. NGS enables the concurrent production of a large number of short DNA sequences (100–250 nucleotides), which may then be arranged and compared with existing genomes [ ]. Currently, there are many prominent NGS systems available for purchase. These include Illumina’s Genome Analyzer, HiSeq 2000, and MiSeq models; Life Sciences’ SOLiD, PGM, and Proton models; Roche’s GS-FLX and GS Junior models; Helicos Biosciences’ Helioscope model; and Pacific Biosciences’ SMRT system. Each of these options provides high-resolution and cost-effective digital information on DNA sequences. The consensus is that tumours arise from genomic mutations, which may be effectively identified by NGS. Point mutations, small insertions or deletions (InDels), changes in the number of copies of a DNA segment (CNV), chromosomal rearrangements, and epigenetic modifications are some of these mutations [ ]. NGS has emerged as the preferred technique for detecting somatic cancer genome mutations and is transforming the approach to studying cancer genomes [ ].
8
Genetic markers of prostate cancer
Genomic analysis is extensively used for the investigation of illness biomarkers. It focuses on germ-line genetic markers, which are stable throughout an individual’s lifetime and may be examined at any age [ ]. Medical professionals use several variables to assess the prognosis of prostate cancer, including the D’Amico criteria, which take into account tumour stage, Gleason score, and PSA levels [ ] and additional prognostic indicators include PSA density and the number of positive biopsy cores [ ]. Obtaining a precise prognosis is crucial since the treatment options, ranging from observation to various therapeutic approaches, are strongly influenced by it. Hence, scientists choose molecular markers to more effectively classify patients and ascertain the need for therapy, its magnitude, and the need for before or post-treatment evaluations.
Genome-wide association studies have shown many genetic polymorphisms and inherited variations that are linked to susceptibility to prostate cancer. In addition, the urine-based tests Select MDx, Mi-Prostate Score, and ExoDx have offered valuable information for identifying patients who might potentially benefit from a prostate biopsy [ ]. Prostate Cancer Antigen 3 (PCa3) and Confirm MDx accurately predicted the results of future biopsies in men who had previously had negative pathology findings [ ]. According to a study by Farha and Salami [ ], commercially accessible tools such as Decipher, Oncotype DX, and Prolaris have been shown to enhance the categorization of prostate cancer risk. These techniques are effective in identifying men who are at the greatest risk of experiencing negative outcomes [ ]. AR-V7 expression is a reliable indicator of resistance to abiraterone and enzalutamide while on the other hand, metastatic patients with mutations in DNA mismatch repair genes may benefit from treatment with a poly (ADP-ribose) polymerase-1 inhibitor or platinum-based chemotherapy [ ].
Also, Gen-Probe offers the biomarker prostate cancer antigen 3 (PCA3) as a commercially available diagnostic tool [ ]. This noncoding RNA is only located in the prostate and may be detected in urine and prostatic fluid. While it does not require blood collection, it is more intrusive than blood testing since it requires a digital massage of the prostate before taking a urine sample. PCA3 overexpression is seen in around 95% of prostate cancer patient biopsies, as opposed to samples from healthy individuals or those with benign prostatic hyperplasia. This detection method has a good specificity rate [ ].
9
Epigenetic markers of prostate cancer
Epigenetic changes and genetic abnormalities play a crucial role in the formation and advancement of cancer. The alterations mentioned include DNA methylation, microRNA, and histone modifications, which together make up the epigenome [ , , ]. The role of DNA mutations in the development of cancer has been well recognised. Recent research has shown a strong connection between cancer and epigenetic changes, which are modifications to DNA that do not entail changes to the DNA sequence. Epigenetic alterations include miRNA silencing, histone modifications, and DNA methylation, with the latter being the most prevalent and comprehensively studied kind of epigenetic alteration. In humans, this process entails the addition of a methyl group (CH3) to a pair of nucleotide bases, particularly when a cytosine (C) comes after guanine [ ].
The association between DNA methylation of cytosine at the N5 position in CpG sequences and the development of cancer is well established. In cancer, there is a significant reduction in methylation across the whole genome, along with an increase in methylation at some genes, which causes them to become inactive at the transcriptional level [ ]. Genes such as INK4a, RASSF1a, and APC have tumour suppressor activities that are deactivated as a result of hypermethylation occurring at their CpG islands. The molecular catalysis performed by DNMTs (DNA methylases) might potentially serve as a first indication of cancer progression that is associated with the process of ageing. The absence of GSTpi methylation was seen in normal epithelium, whereas proliferative inflammatory atrophy showed a methylation rate of 6.4%. High-grade PIN exhibited a methylation rate of 70%, and prostate cancer had the highest methylation rate of 90% [ ]. When the methylation of the APC gene was taken into account combined with the methylation of GSTpi, the ability to diagnose cancer achieved a remarkable accuracy of 100% [ ].
10
Treatment of prostate cancer
10.1
Surgery
In the context of prostate cancer in males, surgery is seen as a component of multimodality approaches rather than a standalone treatment [ ] recommend surgery as the usual course of action for locally advanced prostate cancer that poses a high risk. The most commonly used surgical interventions for prostate cancer are pelvic lymphadenectomy and radical prostatectomy [ ]. Due to the detrimental consequences such as a significant proportion of positive surgical margins, a potential for lymph node metastases, and a substantial likelihood of PSA recurrence, radical prostatectomy has conventionally been opposed for patients with high-risk prostate cancer. Research has shown that surgery is more effective than cautious observation in reducing mortality, the risk of local progression, and the risk of metastasis [ ].
10.2
Radiation therapy
Radiotherapies are considered the second most prominent treatment method for treating localised high-risk prostate tumours, behind radical prostatectomy. Brachytherapy and external-beam radiation are widely used treatment options for prostate cancer that have made substantial progress in both clinical and technical aspects in recent years [ ]. Low-dose rate brachytherapy is a procedure where radioactive seeds with a half-life of 60 days are implanted using ultrasound guidance [ ].
10.3
Proton beam therapy
Radiotherapies are considered the second most prominent treatment method for treating localized high-risk prostate tumors, behind radical prostatectomy. Brachytherapy and external-beam radiation are frequently used treatment methods for prostate cancer that have had notable progress in both clinical and technical aspects in recent years [ ]. Low-dose rate brachytherapy is a procedure where radioactive seeds with a half-life of 60 days are implanted using ultrasound guidance [ ].
10.4
Cryosurgery
Cryosurgery, a therapeutic technique, employs extreme cold to eliminate abnormal or injured tissue, such as prostate tumours. This technique employs liquid nitrogen as the refrigerant, which is then applied to the diseased tissue in the form of a supercooled liquid spray. Targeted cryotherapy has emerged as a less brutal alternative for treating locally progressed low-risk prostate cancer, and it is unquestionably a captivating concept [ ].
10.5
Hormonal therapy
Morgentaler [ ] states that androgens are the primary source of fuel for prostate tumours. Dihydrotestosterone, the primary intracellular form of testosterone, accounts for almost 90% of the overall androgenic activity in the body [ ]. The AR is a ligand-dependent transcription factor that operates inside the cell nucleus [ ]. Despite structural and metabolic differences, Dihydrotestosterone is a very powerful androgen that binds to the AR with a comparable affinity to testosterone [ ]. Normal levels of adrenal androgens have little effect on the prostate. While androgen activation of the AR is the primary mechanism for promoting prostatic growth, prostate cancer may also use other routes. Prostate cancer may develop when these pathways can activate, amplify, enhance, or bypass the androgen receptor without the need for androgen stimulation [ ]. Metastatic prostate cancer is often managed by androgen deprivation treatment involving the use of drugs majorly the luteinizing hormone-releasing hormone agonists and antagonists that reduce androgen signaling (Polotti et a/., 2017; [ ]). This androgen deprivation treatment procedures provides prompt and substantial therapeutic advantages for men suffering from symptomatic metastatic prostate cancer [ ].
10.6
Chemotherapy
Chemotherapy is typically considered ineffective for treating prostate cancer. Before the mid-twentieth century, there was a prevailing belief that chemotherapy was not useful in treating prostate cancer [ ]. However, after that time, the use of chemotherapeutic in patients with hormone-refractory prostate cancer has considerably decreased pain and enhanced quality of life while simultaneously decreasing PSA levels [ ]. Chemotherapy drugs such as mitoxantrone, doxorubicin, vinblastine, paclitaxel, docetaxel, and others are often used to treat advanced prostate cancer [ ].
10.7
Dietary strategies
The development of cancer is attributed to the interplay between an individual’s genetic predisposition, their lifestyle history, including factors such as diet, and several other circumstances. Dietary alteration plays a vital role in cancer prevention because some dietary components may lower the risk of cancer while others can raise the risk [ ]. Fig. 2 below presents the schematics on the different treatments of prostate cancer ( Fig. 3 ).
