Highlights
- •
Natural products are well-studied for prostate cancer prevention but less for therapy.
- •
Natural products are used to complement conventional therapies in prostate cancer.
- •
Natural products show promise in preclinical studies but limited efficacy in trials.
- •
Translating preclinical findings into clinical practice remains a challenge.
- •
Well-designed trials are needed to assess toxicity and efficacy of natural products.
Abstract
Prostate cancer is the most commonly diagnosed cancer and the second leading cause of cancer-related death among men in the United States. The global burden of this disease is rising, placing significant strain on healthcare systems worldwide. Although definitive therapies like surgery and radiation are often effective, prostate cancer can recur and progress to castration-resistant prostate cancer in some cases. Conventional treatments for prostate cancer often have substantial side effects that can greatly impact patients’ quality of life. Therefore, many patients turn to complementary therapies to improve outcomes, manage side effects, and enhance overall well-being. Natural products show promise as complementary treatments for prostate cancer, offering anticancer properties with a low risk of adverse effects. While preclinical research has produced encouraging results, their role in prostate cancer treatment remains controversial, largely due to inconsistent and limited success in clinical trials. This review explores the mechanisms of action of key natural products in prostate cancer management and summarizes clinical trials evaluating their efficacy and safety. It underscores the need for high-quality, rigorously designed, and adequately powered studies to validate the therapeutic potential and safety of these supplements in cancer care. Additionally, we propose future directions to enhance their role in addressing the complex challenges associated with prostate cancer.
1
Introduction
Prostate cancer (PCa) is the most common cancer among men in the United States and the second most common globally, with an estimated 1.5 million new cases in 2022 alone. It is the second leading cause of cancer-related mortality among men in the United States and the fifth worldwide [ , ]. Developed countries have nearly 3 times the incidence rate of PCa compared to developing countries, with the highest rates found in Northern Europe, North America, Australia, New Zealand, and the Caribbean, and the lowest rates in various Asian and African regions [ ]. This discrepancy in incidence is complex and multifactorial, involving a combination of factors including genetics, demographic differences, lifestyle choices, healthcare access, and environmental exposures. As different cultures and developing countries industrialize and adopt Western lifestyles, their cancer rates are beginning to rise. For instance, Japanese men, who traditionally have a lower PCa risk, develop risks similar to Americans after immigrating to the United States. Furthermore, as successive generations of men of Asian descent reside in Western countries, their PCa incidence and mortality rates tend to increase [ ]. The Western diet—characterized by high consumption of red meat, processed foods, fat, and sugar, along with low intake of fruits and vegetables—has been associated with an increased risk of PCa [ ]. Since diet is a modifiable risk factor, a better understanding of the role of nutrients in PCa can lead to strategies that optimize nutrition and supplementation, potentially playing a crucial role in both prevention and treatment of the disease.
Most patients diagnosed with localized PCa typically undergo radical prostatectomy and/or radiation therapy. While many achieve long-term remission or cure, 20%–40% will experience a recurrence [ ]. The disease state referred to as biochemical recurrence is defined as prostate-specific antigen (PSA) recurrence without evidence of disease on conventional imaging [ ]. Within five years of their initial diagnosis, approximately 10%–20% of patients will develop metastatic castration-resistant PCa (mCRPC), which has a poor prognosis [ ]. The treatment options aimed at improving overall survival often come with significant adverse effects and can negatively impact quality of life, leading to early treatment discontinuation and poor adherence. Consequently, the use of complementary and integrative medicine in PCa treatment has gained significant popularity, with nearly 50% of patients using at least 1 such modality during their disease course [ ].
While numerous preclinical and clinical studies have highlighted the potential use of natural products in reducing the risk of PCa, their benefits in PCa treatment have been less extensively explored. For this paper, we conducted a literature review through PubMed with over 2,000 articles screened. This review concentrates on the potential of natural products as complementary or integrative treatments rather than preventive measures. It examines the anticancer properties of key natural products, including vitamins C, D, and E, selenium, zinc, curcumin, lycopene, plumbagin, green tea polyphenols, soy isoflavones, pomegranate, resveratrol, and omega-3 fatty acids, and evaluate their efficacy in clinical trials over the years and across the different clinical stages of prostate cancer ( Fig. 1 ). Furthermore, we discuss protein intake and its role in influencing PCa risk and progression.
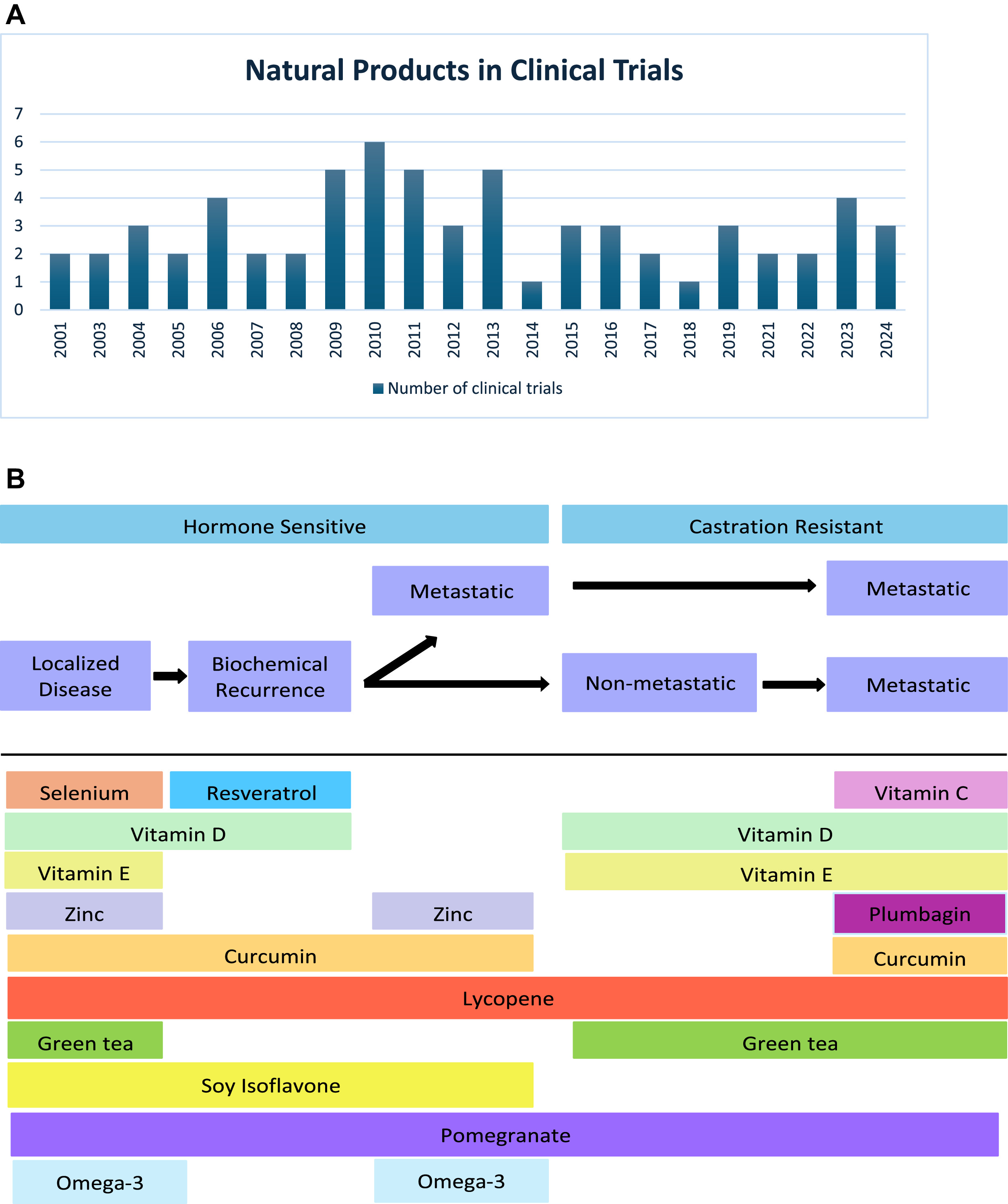
2
Vitamins and minerals
2.1
Vitamin C
Vitamin C (ascorbate or ascorbic acid) is a water-soluble vitamin that is an essential nutrient for humans. Citrus fruits, berries, tomatoes, potatoes, and leafy greens are rich reservoirs of vitamin C [ ]. Vitamin C has shown promise in preclinical and small clinical trials across different cancers, including ovarian and pancreatic cancers [ ].
The function of vitamin C varies based on the dosage and mode of administration. At low doses, it serves as an antioxidant, while at high doses, it functions as a prooxidant [ , ]. Pharmacologic concentrations of vitamin C, which can only be achieved through intravenous (IV) administration rather than oral dosing, have been shown to be selectively toxic to cancer cells. The predominant mechanism through which pharmacologic vitamin C exerts its anticancer effect is via the generation of extracellular hydrogen peroxide [ , ]. These reactive oxygen species (ROS) diffuse intracellularly and induce oxidative stress in cancer cells through their downstream effects, including DNA and mitochondrial damage and apoptotic pathway stimulation [ ].
Prospective clinical trials investigating the use of vitamin C in the treatment of PCa are limited, and thus far none have demonstrated efficacy. A single-arm, phase II study reported that high-dose IV vitamin C (60g) given once weekly for 12 weeks failed to produce a PSA50 response rate (≥50% decline in PSA level from baseline) or disease remission in patients with chemotherapy-naive mCRPC [ ]. Similarly, a recent randomized, placebo-controlled, phase II trial found that high-dose IV vitamin C combined with docetaxel did not improve the PSA response rate, progression free survival, or overall survival, or mitigate docetaxel toxicity in patients with mCRPC [ ]. An ongoing phase II trial is investigating the combination of the PARP inhibitor olaparib and IV vitamin C in patients with mCRPC ( Table 1 ) [ ].
Disease State | Trial ID | Study Title | Status | Target Population | Intervention | Design | Mean Intervention Duration | Primary Objective |
---|---|---|---|---|---|---|---|---|
Localized PCa | NCT02064673 | Randomized Trial of Adjuvant Curcumin After Prostatectomy | Recruiting | Status post RP | Curcumin 500 mg PO BID | Curcumin vs. Placebo | 6 months | Recurrence-free survival |
NCT03769766 | A Randomized, Double-Blind, Placebo-Controlled Trial of Curcumin to Prevent Progression of Biopsy Proven, Low-risk Localized Prostate Cancer Patients Undergoing Active Surveillance | Recruiting | Low risk, localized PCa | Curcumin 500 mg PO BID | Curcumin vs. Placebo | 24 months | Rate of disease progression | |
NCT04731844 | Efficacy of Curcumin and Piperine in Patients on Active Surveillance for Either Monoclonal Gammopathy of Unknown Significance (MGUS), Low-risk Smoldering Multiple Myeloma (SMM) or Early Stage Prostate Cancer: A Pilot Study | Recruiting | Non-metastatic PCa on AS | Curcumin plus Piperine 4g/5mg PO BID | Open-label, uncontrolled | 12 months | Response rate | |
NCT04300855 | Phase II Clinical Trial of Green Tea Catechins in Men on Active Surveillance (AS) | Recruiting | Low to intermediate grade PCa on AS | Sunphenon® 90D (405mg EGCG) 3 capsules BID | Sunphenon® 90D vs. placebo | 24 months | Rate of Progression to PCa | |
NCT04597359 | A Phase II Randomized Double Blinded Study of Green Tea Catechins (GTC) vs. Placebo in Men on Active Surveillance for Prostate Cancer: Modulation of Biological and Clinical Intermediate Biomarkers | Recruiting | Very low, low and favorable intermediate risk PCa on AS | Green tea catechins PO BID | Green tea catechins vs. placebo | 6 months | Change in the highest percent Ki-67 expression | |
NCT02176902 | A Phase II Randomized, Open-Label, Two-Arm Study of a Low Omega-6, High Omega-3 Fat Diet Combined with Fish Oil vs. a Control Group in Men on Active Surveillance for Prostate Cancer | Active, not recruiting | PCa on AS | Dietary counseling, guidelines for low-fat diet and 4 omega-3 fatty acid capsules PO | Dietary counseling and fish oil vs. no intervention | 12 months | Ki-67 index | |
NCT03824652 | WALNUTS for POWER: Polyphenols, Omega-3 Fatty Acids, Weight Loss, and EneRgy | Recruiting | PCa scheduled to undergo RP | 2 ounces of walnuts daily and weekly phone counseling with dietitian | Usual diet with walnuts and dietary counseling vs. usual diet | 4-10 weeks | Mean difference in prostatic tissue Ki-67 expression from baseline biopsy to RP | |
NCT02333435 | Effects of EPA on Prostate Cancer Cells Proliferation and Quality of Life (RCT-EPA) | Active, not recruiting | PCa scheduled to undergo RP | 3g/day of purified eicosapentanoic acid (EPA) capsules | Purified EPA vs. placebo | 14 months | Change in PCa Proliferative Index | |
NCT02759380 | Can Dietary Phytoestrogens Slow Down Prostate Tumor Proliferation? A Randomized Study (PRODICA) | Recruiting | Intermediate risk-level PCa scheduled for RP | Package containing food with high amounts of phytoestrogens (≥100mg isoflavonoids and ≥100mg lignans) consumed daily. Food package contains 30g of flaxseed, 25g of roasted soybeans and 50g of frozen green edamame beans | Phytoestrogen-rich foods and general nutritional counseling vs. general nutritional counseling | Until day before RP (at least 6 weeks) | Percentage of positive stained cells with Ki-67 | |
NCT01823562 | Dietary Fruit Bioactives and Prostate Cancer | Active, not recruiting | PCa scheduled to undergo RP | All arms for 4-6 weeks followed by RP: ARM I: regular diet ARM II: low polyphenol diet ARM III: low ellagitannin diet ARM IV: low ellagitannin diet and receive lower-dose black raspberry gummy PO daily ARM V: low ellagitannin diet and receive higher-dose black raspberry gummy PO daily ARM VI: low ellagitannin diet and receive lower-dose black raspberry nectar PO daily ARM VII: low ellagitannin diet and receive higher-dose black raspberry nectar PO daily | Regular diet vs. low polyphenol diet vs low ellagitannin diet vs. lower-dose lyophilized black raspberry gummy vs. higher-dose black raspberry gummy vs. lower-dose black raspberry confection vs. higher-dose black raspberry confection | 4-6 weeks | Determine safety and compliance of varying lyophilized black raspberry delivery vehicles (a gummy confection and a nectar) at 2 different doses | |
NCT03665922 | Biomarkers of Sulforaphane/Broccoli Sprout Extract in Prostate Cancer | Active, not recruiting | PCa scheduled to undergo RP | 8 BroccoMax® tablets (64mg of sulforaphane) | BroccoMax® tablets vs. placebo | 4 weeks | Change in serum levels of palmitic acid (primary free fatty acid) | |
NCT05590624 | Elucidating the Metabolic Impact of Isocaloric, Controlled, Mediterranean-Type Diets in Treatment-Naïve Men with Prostate Cancer on Active Surveillance (DINE Study) | Not yet recruiting | Localized, treatment-naïve PCa on AS | Arm 1: Dietary Intervention #1=Low Fat Mediterranean followed by Dietary Intervention #2=Lower Carbohydrate Mediterranean. Arm 2: Dietary Intervention #1=Lower Carbohydrate Mediterranean followed by Dietary Intervention #2=Low Fat Mediterranean | Low fat Mediterranean diet followed by lower carbohydrate Mediterranean diet vs lower carbohydrate Mediterranean diet followed by low fat Mediterranean diet | 2 weeks per dietary intervention | Impact of Mediterranean diets on non-malignant prostate tissue metabolism | |
BCR | NCT03087903 | A Phase 2 Study of Grape Seed Extract (GSE) Product in Asymptomatic Non-Metastatic Prostate Cancer Patients with Rising Prostate Specific Antigen (PSA) | Active, not recruiting | Asymptomatic non-metastatic PCa with rising PSA | 150mg of Grape Seed Extract product PO BID | Open-label, uncontrolled | 12 months | Number of patients with PSA response (increase in PSADT of 30%) |
NCT03753334 | Effects of EPA in Men with Biochemical Recurrence or Progression of Prostate Cancer (RCT-EPAII-BCR) | Recruiting | BCR or PCa progression after curative treatment | 5g/day of omega-3-rich fish oil including 4g of purified monoglycerides EPA capsules, daily | Omega-3-rich fish oil vs. placebo | 12 months | Change in PSADT from baseline to 12 months | |
mHSPC | NCT05802121 | The Role of Akkermansia Muciniphilia in Combating the Metabolic Effects of Androgen Deprivation Therapy in Men with Metastatic Prostate Cancer | Not yet recruiting | Metastatic castration-sensitive PCa | 6 apple cider vinegar pills, enteric coated (equivalent of 500 mg/capsule containing 25 mg acetate x 6 capsules) daily | Apple cider vinegar vs. placebo | 3 months | Increase in proportion of A. muciniphilia in stool samples, and tolerability and side effects |
mCRPC | NCT05501548 | Phase II Study of PARP Inhibitor Olaparib and IV Ascorbate in Castration Resistant Prostate Cancer | Recruiting | Metastatic castration-resistant PCa | Olaparib 300mg PO BID and ascorbic acid 1g/kg IV twice weekly | Open-label, uncontrolled | Up to 5 years | PSA50 response (50% reduction in PSA from baseline) |
Multiple PCa Disease States | NCT04519879 | A Randomized Phase 2 Trial of White Button Mushroom Supplement in Patients with Biochemically Recurrent Prostate Cancer Following Local Therapy and in Therapy Naive Patients with Favorable Risk Prostate Cancer Undergoing Active Surveillance | Recruiting | BCR (cohort 1) or therapy- naive patients with favorable risk prostate cancer undergoing AS (cohort 2) | ARM IA: white button mushroom extract PO BID on day 1. Treatment repeats every 4 weeks for cycles 1-3 then every 12 weeks for cycles 4-6 (36 weeks) ARM IB: clinical observation for 12 weeks ARM IIA: white mushroom extract PO BID on day 1 Treatment repeats every 12 weeks for 4 cycles (48 weeks) ARM IIB: active surveillance for 48 weeks | Arm I: white mushroom extract vs. clinical observation Arm II: white mushroom extract vs. active surveillance | 36-48 weeks | Cohort 1: PSA (ng/mL) levels Cohort 2: Relative change in PSA |
NCT05471414 | Whole-Food Plant-Based Diet (WFPBD) to Control Weight and Metabo-Inflammation in Overweight/Obese Men with Prostate Cancer Receiving Androgen Deprivation Therapy (ADT): A Multi-Center Randomized Control Trial | Recruiting | PCa on ADT or underwent bilateral orchiectomy | Pre-packaged, plant-based meals delivered weekly for 8 weeks followed by 18 weeks of continued coaching, but no pre-packed meals | Whole-food, plant-based diet vs. general nutritional counseling | 26 weeks | Change in weight from baseline to 4 weeks post-randomization | |
NCT03580499 | A Pilot Study of the Effects of Vitamin B6 on Hot Flash Symptoms in Prostate Cancer Patients | Active, not recruiting | PCa on ADT | Vitamin B6 PO daily | Open-label, uncontrolled | 12 weeks | Vitamin B6 in improving frequency/severity of hot flashes | |
NCT05838716 | High-dose Vitamin D Supplementation for ADT-Induced Bone Loss in Older Prostate Cancer Patients | Recruiting | PCa on ADT without bone metastases | High-dose vitamin D PO | High-dose vitamin D vs. placebo | 52 weeks | Effect of vitamin D on ADT-induced bone mineral density loss in total hip, femoral neck, distal radius, and lumbar spine (L1-L4) | |
NCT06172283 | A Pilot, Feasibility Study of Intermittent Fasting in Prostate Cancer Patients Receiving Androgen Deprivation Therapy | Recruiting | PCa on ADT | Intermittent Fasting: 16h periods of fasting everyday | Open-label, uncontrolled | 3 years | Feasibility of intermittent fasting | |
NCT02946996 | Randomized Phase II Study of Pharmacologic Manipulation of AGE (Advanced Glycation End products) Levels in Prostate Cancer Patients Receiving Androgen Deprivation Therapy | Active, not recruiting | PCa on ADT | 1 capsule of OPC (derivative of grape seed extract) BID | Open-label, uncontrolled | 12 weeks | AGE (Advanced Glycation End products) level reduction | |
NCT03496805 | A Phase 2 Double-blind, Placebo-controlled Study of the Effects of Muscadine Grape Extract in Men with Prostate Cancer on Androgen Deprivation Therapy | Recruiting | PCa on ADT | Muscadine grape extract 4 capsules PO BID | Muscadine grape extract vs. placebo | 12 months | Compare levels of fatigue |
2.2
Vitamin D
The fat-soluble vitamin D3 (cholecalciferol) is synthesized in the human skin from 7-dehydrocholesterol upon exposure to sunlight or obtained from dietary sources such as fatty fish and fish oils. Vitamin D can also be ingested in the form of vitamin D2 (ergocalciferol) from plant-based sources. Regardless of its origin, vitamin D undergoes two hydroxylation reactions in the body to be converted into its biologically active form, 1,25(OH) 2 vitamin D, also known as calcitriol [ ].
The antitumor effects of vitamin D are thought to be mediated in part through its interaction with the vitamin D receptor, which is present on human PCa cells [ ]. Upon binding to its receptor, calcitriol blocks G1 to S cell cycle phase transition, increases the levels of cyclin-dependent kinase (CDK) inhibitors p27Kip1 and p21Waf1/Cip1, decreases CDK2 activity, decreases phosphorylation of the retinoblastoma (Rb) protein, and represses E2F transcriptional activity in LNCaP cells [ , ]. Calcitriol reduces the angiogenic signaling molecule, angiopoietin-2, decreases the levels of anti-apoptotic proteins like Bcl-2 and Bcl-xL, and stimulates the mitochondrial release of cytochrome c [ , ]. Calcitriol was found to augment the efficacy of various cytotoxic chemotherapy agents in multiple preclinical tumor models [ ].
Evidence suggests that sun exposure reduces the risk of PCa [ ], and low levels of vitamin D increase the risk [ ]. A study by Zheng et al.[ ] showed that vitamin D deficiency promotes PCa growth in bone by altering the bone microenvironment. Numerous clinical trials studying the potential role of vitamin D in the treatment of PCa have yielded conflicting results ( Table 2 ) [ ]. In the context of localized PCa or biochemical recurrence, vitamin D shows promising therapeutic potential, although clinical trials in this area are still limited [ , , , ]. Further research is needed to conclusively establish its beneficial effects in this space. Vitamin D has been extensively studied in the mCRPC setting, but it has not demonstrated efficacy, whether used alone, in combination with chemotherapy agents, or with dexamethasone [ , , , , , ]. Consequently, its use is not supported as treatment in the mCRPC space.
First Author | Type of Study | Patients | Intervention | Intervention Duration | Results |
---|---|---|---|---|---|
Osborn[ ] | Phase II | n = 13 patients with mCRPC | Daily oral calcitriol dose of 0.5μg increasing to a maximum dose of 1.5μg | Duration of calcitriol until disease progression or toxicity | No objective response was observed. There was a 25% and 45% PSA decrease observed in 2 patients. |
Gross[ ] | Open-label, pilot study | n = 7 patients with BCR | Daily oral calcitriol dose of 0.5μg increasing to a maximum dose of 2.5μg | 6 to 15 months | Rate of PSA rise significantly decreased. |
Liu[ ] | Phase I | n = 25 patients with mCRPC | In 5 cohorts, daily oral 1α-Hydroxyvitamin D2 was administered ranging from 5μg to 15μg | 110 total courses of were administered | 1α-Hydroxyvitamin D2 was well tolerated with main toxicities being hypercalcemia and renal insufficiency, which were reversible with drug discontinuation. |
Liu[ ] | Phase II | n = 26 patients with mCRPC | Daily oral 1α-Hydroxyvitamin D2 dose 12.5μg | 20 patients completed ≥ 8 weeks of therapy | No objective responses were observed. Six (30%) evaluable patients had stable disease for >6 month. |
Morris[ ] | Phase I | n = 31 patients with progressive prostate cancer | In cohorts of 3-6 patients, calcitriol was administered for 3 days per week, starting at a dose of 4μg/day up to 30μg/day. IV zoledronate (4mg) monthly | Median duration was 12 weeks (range: 6–84 weeks) | Calcitriol was well tolerated at all doses 3 times per week with monthly IV zoledronate. |
Beer [ ] | Phase II | n = 17 patients with mCRPC | High-dose oral calcitriol (0.5μg/kg) in combination with IV carboplatin given once every 4 weeks | Median duration was 13 weeks (range: 3–44 weeks) | Oral calcitriol combined with carboplatin did not increase the PSA50 response rate when compared to previously reported activity of carboplatin alone |
Tiffany [ ] | Phase I/II | n = 24 patients with mCRPC | 60μg calcitriol orally on day 1, 280mg estramustine orally 3 times daily on days 1 to 5 and 60mg/m docetaxel on day 2 (70mg/m after cycle 1) every 21 days for up to 12 cycles | Median duration was 15 weeks (range: 3–36 weeks) | Oral calcitriol combined with docetaxel plus estramustine did not increase toxicity. PSA and measurable disease response rates in the chemotherapy-naïve patients were similar to previously reported rates of docetaxel plus estramustine; however, the study was not designed to determine treatment efficacy. |
Schwartz[ ] | Phase I/II | n = 18 patients with CRPC | Vitamin D analogue, 19-nor-1a-25-dihydroxyvitamin D2 (paricalcitol) IV 3 times per week on an escalating dose of 5 to 25μg (3-15μg/m2) | 11 patients were dose escalated, for a total of 29 cycles | No PSA50 response was observed. |
Trump [ ] | Phase II | n = 43 patients with CRPC | 4mg of dexamethasone 4 times weekly combined with oral calcitriol 3 times weekly at 8μg for 1 month, 10μg for 1 month, and 12μg thereafter | Average time on study was about 127 days | Eight patients (19%) experienced a PSA50 response rate, but it did not appear higher than expected with dexamethasone alone |
Flaig [ ] | Phase II | n = 34 patients with CRPC | 1mg oral dexamethasone and 0.5μg calcitriol daily. Carboplatin initially given weekly, but the protocol was later changed to carboplatin for 4 weeks of a 6-week cycle | NA | Combination of dexamethasone, calcitriol, and carboplatin produced a PSA response in 13 out of 34 patients (38.2%). However, the predetermined statistical standard for success set for the trial was not met, which required a PSA response in 15 of 34 patients. Median overall survival was 97.7 weeks |
Beer [ ] | Double-blind randomized phase II | n = 250 patients with progressive mCRPC | Weekly docetaxel 36mg/m2 IV for 3 weeks of a 4-week cycle combined with either 45μg DN-101 (high-dose calcitriol) or placebo taken orally 1 day before docetaxel | Median duration was 154 days for DN-101 and 155 days for placebo | The addition of DN-101 to weekly docetaxel did not produce a statistically significant improvement in the PSA response rate with 63% in DN-101-treated patients and 52% for placebo-treated patients. The study suggests that the addition of DN-101 to docetaxel was associated with improved survival with a reduction in the risk of death by approximately one third. |
Attia[ ] | Double-blind randomized phase II | n = 70 patients with mCRPC | 4-week cycle, docetaxel (35mg/m2 IV, days 1, 8, and 15) with doxercalciferol (10μg orally) or placebo | Median duration for doxercalciferol was 24 weeks (range: 4–60 weeks) and for placebo was 24 cycles (range: 4–48 weeks) | Daily doxercalciferol with weekly docetaxel did not enhance PSA response rate or survival. |
Srinivas [ ] | Phase II | n = 21 patients with BCR after local therapy | DN101 (45μg once per week) and naproxen (375mg twice daily) for one year | 1 year | Calcitriol and naproxen had overall good tolerability and PSADT prolongation in two-thirds of the patients. |
Chadha [ ] | Phase II | n = 18 patients with CRPC | 4mg oral dexamethasone twice weekly and IV calcitriol (74μg) once weekly | Median duration was 13.5 weeks (range: 5–61 weeks) | High-dose, weekly, IV calcitriol in combination with dexamethasone was well tolerated but failed to produce a clinical or PSA response. |
Scher [ ] | Randomized, Open-Label Phase III | n = 953 patients mCRPC | ASCENT treatment arm: 45μg DN-101, 36mg/m2 docetaxel, and 24mg dexamethasone weekly for 3 of every 4 weeks vs. control arm: 5mg prednisone twice daily with 75mg/m2 docetaxel and 24mg dexamethasone every 3 weeks | Duration of docetaxel up to 30 weeks or until unacceptable toxicity or clinical disease progression | The DN-101 and docetaxel treatment was associated with a shorter survival than standard of care docetaxel, which could be attributable to the weekly docetaxel dosing. |
Marshall [ ] | Open-label | n = 52 patients with low-risk prostate cancer on active surveillance | Vitamin D3 soft gels (4000 IU) | One year | Repeat biopsy showed a decreased number of positive cores in more than half of the patients. No significant changes in PSA levels were observed. |
Wagner [ ] | Double-blind randomized phase II | n = 66 patients with localized prostate cancer (Gleason 6 or 7) scheduled to undergo radical prostatectomy | Random allocation to 1 of 3 vitamin D3 doses: 1. 400IU (10μg), 2. 10,000IU (250μg), and 3. 40,000IU (1000μg) orally once per day for a 3- to 8-week ending the day before radical prostatectomy | Mean duration was 33.6 ± 9.5 days | Prostate tissue and serum levels of vitamin D metabolites, including calcitriol, increased dose dependently and were significantly higher in the 40,000-IU/d group. There was a modest reduction in the PSA levels with higher vitamin D doses (10,000 and 40,000 IU/day) and no signs of toxicity. |
However, patients with PCa metastatic to the bone should be monitored for vitamin D levels, with supplementation provided when deficiencies are detected in order to prevent bone-related complications. It is important to keep vitamin D levels in the normal range, particularly in those undergoing androgen-deprivation therapy (ADT), which can cause loss of bone mineral density (BMD) and increased risk of fracture [ ]. A study showed that ADT-induced BMD loss was greatest in the lumbar spine within the first year of use. However, vitamin D supplementation was associated with improved BMD in the lumbar spine during this first year [ ]. According to the National Comprehensive Cancer Network (NCCN) guidelines, all patients receiving ADT should receive 1000–1200mg of calcium daily from food or supplements and maintain serum vitamin D3 levels of 30 to 50 ng/mL [ ]. An ongoing trial is evaluating the use of high-dose vitamin D supplementation for ADT-induced bone loss in patients with early-stage PCa ( Table 1 ). In a 2024 phase II study, high-dose vitamin D (50,000 IU/week) significantly reduced BMD loss in the hip and femoral neck, particularly for patients with low vitamin D baseline levels [ ]. Vitamin D may also improve pain, muscle strength, and quality of life in patients with mCRPC [ ]. Patients with mCRPC and bone metastases are recommended to receive antiresorptive medications, such as denosumab or zoledronic acid, to prevent disease-related skeletal complications [ ]. In these patients, maintaining normal serum vitamin D levels is particularly important, as there is an increased risk of hypocalcemia with these medications, especially in cases of vitamin D deficiency.
2.3
Vitamin E
Vitamin E is a fat-soluble antioxidant that can be divided into two major groups, tocopherols and tocotrienols, with each group consisting of four isomers: alpha, beta, gamma, and delta. Vitamin E is found in plant-based oils, nuts, and seeds, with wheat germ oil being the richest source of tocopherols, specifically α and β. Significant amounts of vitamin E can also be found in fruits and vegetables, including blackberries, avocado, broccoli, and asparagus [ ].
Tocotrienols, particularly the gamma and delta isoforms, have been demonstrated to be more effective than tocopherols in inhibiting PCa cell growth in androgen-independent PC-3 cells [ ]. Gamma-tocotrienol (γ-T3) induces PCa cell apoptosis through the suppression of NF-kB activity, epidermal growth factor receptor (EGFR) expression, and Id1 and Id3 expression [ ]. γ-T3 promotes PCa cell apoptosis through the activation of the JNK-signaling pathway and inhibits PCa cell invasion through increased expression of E-cadherin and γ-catenin [ ]. Since docetaxel induces apoptosis under hypoxic conditions by activating the JNK2/PHD1 signaling pathway [ ], the addition of γ-T3 to docetaxel enhances its effect in vitro and in vivo, suggesting a synergistic effect against PCa cells [ , ]. A tocotrienol-rich fraction extracted from palm oil selectively induces a G0/G1 cell cycle arrest and accelerates apoptotic events in PCa cells [ ]. γ-T3 downregulates the pro-angiogenic factor angiopoietin-1 (Ang-1) expression suppressing the Ang-1/Tie-2 signaling pathway in PCa cells [ ].
While the effect of vitamin E on PCa risk has been well studied, with the physicians health study reporting no risk reduction [ ] and the SELECT trial reporting increased risk [ ], its role in the treatment of PCa has rarely been studied in clinical trials. A pilot phase 0 trial determined that 2-week supplementation with high-dose γ-tocopherol-rich vitamin E increased the prostate levels of γ- and δ-tocopherols but had no significant effect on PSA reduction in patients with PCa undergoing radical prostatectomy [ ]. A phase I/IIa dose-escalation study was conducted to evaluate the effects of oral APC-100 (2,2,5,7,8-pentamethyl-6-chromanol), the antioxidant component of α-tocopherol, in patients with CRPC. APC-100 exhibits a dual mechanism of action, functioning both as a potent antioxidant and as an antiandrogen, suggesting its potential efficacy in conditions such as PCa, where oxidative stress and androgens are key factors. The study found that while APC-100 was well tolerated, with PSA stabilization occurring in 25% of patients, the maximum feasibility of the drug was reached with no detectable drug in the plasma [ ].
It will be interesting to evaluate the effects of APC-100 on PCa, if it is reformulated to allow for higher dose escalation. Preclinical studies demonstrate potential synergy between docetaxel and vitamin E through different mechanisms [ , , ]. The addition of γ-T3 and α-tocopherol ether acetate (α-TEA) to docetaxel showed improved effectiveness in reducing cell viability in resistant cells and decreased the amount of docetaxel required [ ]. The combination of γ-T3 and docetaxel lead to a synergistic effect on docetaxel-induced apoptosis [ ]. Well-designed clinical trials are needed to evaluate and validate these findings in patients with PCa. However, based on the current evidence, their implementation in clinical practice cannot yet be recommended.
2.4
Selenium
Selenium is an essential trace element that exists naturally in organic and inorganic forms. The main forms of organic selenium include selenomethionine (SeMet), selenocysteine (SeCys), and methylselenocysteine (MSeC). Inorganic selenium (selenite and selenate) requires conversion into organic forms within the body for utilization. Humans acquire selenium through dietary sources, supplements, and drinking water. Dietary intake of selenium comes from both plant-based foods, such as cereals (the primary source), nuts, vegetables, and fruits, and animal-based foods, including meat, dairy products, fish, seafood, and milk. Selenium can be found in drinking water, primarily as selenate and selenite [ ].
Selenium downregulates androgen receptor (AR) signaling and PSA expression in PCa cells [ ]. In LNCaP cells, selenium induces G1 cell cycle arrest and the upregulation of CDK inhibitors p27Kip1 and p21Cip1 [ ]. Methylseleninic acid (MSeA), a selenium metabolite, reduces hypoxia-inducible factor-1α (HIF-1α) expression and its activity along with its downstream targets, VEGF and GLUT1 [ ]. Selenite induces apoptosis in LNCaP cells, marked by an increase in superoxide production, decrease in mitochondrial membrane potential, release of cytochrome c, and activation of caspases 9 and 3 [ ]. Selenium triggers rapid transcriptional activation of p53, with upregulation of the expression of p53-target genes, such as p21, BAX, and DR5, which plays a causal role in selenium-induced apoptosis [ ]. MSeC downregulates Bcl-2 and upregulates BAD expression in DU145 cells, facilitating apoptosis [ ].
Selenium has been extensively studied for its potential role in PCa chemoprevention, but the results have been largely negative [ , ]. In fact, administering selenium at high doses may increase the risk of aggressive PCa in individuals with certain genetic factors such as the valine (V)/alanine(A) and VV- SOD2 genotype, which are present in 75% of men [ , ]. SOD2 encodes manganese superoxide dismutase (MnSOD), which is the primary mitochondrial enzyme that protects cells from oxidative stress. The AA genotype of SOD2 produces a variant of MnSOD that enables more efficient mitochondrial import and is more active than other genotypes. Among men with the AA genotype, those in the highest quartile of selenium had reduced risk of aggressive prostate cancer [ , ].
The evidence supporting the use of selenium in PCa treatment is limited. In a phase II trial, men with localized, nonmetastatic PCa under active surveillance were administered either 0 µg, 200 µg, or 800 µg of selenium in the form of selenized yeast. Although the trial showed no significant overall impact on PSA velocity, it did find that PSA velocity increased in men who already had high baseline plasma selenium levels [ ]. Furthermore, research by Kenfield et al. [ ] indicated that men with nonmetastatic PCa who supplemented with selenium after diagnosis faced an increased risk of PCa mortality.
Based on these findings, the use of selenium in PCa treatment is not recommended and should be approached with caution due to the potential harmful effects at high doses [ ]. In the SELECT trial, there was a nonsignificant increase risk of type 2 diabetes mellitus in the selenium arm (HR = 1.07) [ ], but in the updated results the HR moved closer to 1 (HR = 1.04) [ ]. However, the potential risk of diabetes with selenium supplementation cannot be excluded, as studies have shown mixed results [ , ].
2.5
Zinc
The primary dietary sources of zinc include red meat, seafood, poultry, grains, and legumes. In the United States, there is inadequate intake of zinc in 20%–25% of adults ages 60 or older [ ]. Interestingly, the normal prostate tissue contains the highest level of zinc compared to any other soft tissue in the human body, a level that significantly declines during the development of PCa [ , ].
Preclinical studies have suggested that zinc supplementation could have therapeutic potential in PCa by restoring normal zinc levels in PCa cells. Physiological levels of extracellular zinc inhibit the growth of LNCaP and PC-3 cells, which is dependent on the ability of PCa cells to accumulate high levels of intracellular zinc [ ]. Physiological levels of zinc induce apoptosis in PCa cells through multiple mechanisms including zinc’s direct effect on BAX-associated pore formation in the mitochondria leading to the release of cytochrome c, which initiates the caspase cascade [ ]. Zinc induces a G2/M cell cycle arrest that is accompanied by the upregulation of p21Waf1/Cip1/Sdi1 mRNA levels [ ].
Findings from the Health Professionals Follow-up Study indicated that long-term, high-dose zinc supplementation (more than 75 mg/day over 15 years) may increase the risk of developing aggressive PCa [ ]. In contrast, the same study found that low-dose zinc supplementation (1–24 mg/day) in patients with nonmetastatic PCa was associated with a lower risk of lethal PCa and all-cause mortality [ ]. Supporting this, a study of dietary zinc intake in a Swedish cohort of PCa patients revealed that higher zinc intake from dietary sources (9–20.1 mg/day) was associated with lower PCa-specific mortality, particularly in patients with localized disease [ ].
Given the association between high-dose, long-term zinc supplementation and the increased risk of developing clinically significant PCa, caution is warranted when considering excessive zinc intake in healthy adult males. However, zinc supplementation as a treatment for PCa remains intriguing and warrants further clinical investigation. In addition, the impaired ability of PCa cells to accumulate and secrete zinc, as indicated by urinary zinc levels, may hold promise as a biomarker for early disease detection [ ].
3
Plant and plant-derived substances
3.1
Curcumin
Curcumin (diferuloylmethane) is the principal bioactive polyphenol of turmeric, which is derived from Curcuma longa rhizomes. Turmeric is widely used as a spice and is the source of curry, with its distinctive yellow color and flavor. It is also used as a coloring agent in butter, cheese, and other food items [ ].
Curcumin treatment leads to the reduction of intracellular testosterone levels in the prostate, thereby preventing AR activation [ ], decreases AR expression at the mRNA and protein level [ ], and downregulates NKX3.1 gene expression [ ]. Curcumin decreases cell proliferation, increases the extent of apoptosis, and inhibits angiogenesis in PCa cells [ ]. Furthermore, curcumin selectively induces apoptosis in PCa cells, which correlates with the downregulation of antiapoptotic proteins, Bcl-2 and Bcl-xL, and activation of caspases 3 and 8 [ ]. The PI3K/AKT signaling pathway is inhibited by curcumin, enhancing apoptosis in PCa cells [ ]. Curcumin also inhibits cell proliferation and tumor progression by inducing the degradation of β-catenin through the regulation of downstream molecules of the Wnt/β-catenin signaling pathway. Since β-catenin is a coactivator of AR transcription, this is an alternative pathway through which curcumin can downregulate AR expression [ ].
Curcumin’s therapeutic efficacy has been studied in numerous clinical trials for a wide range of diseases. A randomized, placebo-controlled trial in patients with PCa undergoing intermittent ADT studied the effect of oral curcumin for 6 months beginning from ADT withdrawal. The overall off-treatment duration of intermittent ADT was not significantly affected, but there were significantly lower PSA progression rates in the curcumin group [ ]. A randomized controlled trial assessed the radio-sensitizing and radio-protective effects of oral curcumin in patients with PCa undergoing radiotherapy. The results showed a reduction in PSA levels to below 0.2ng/ml in both the curcumin and control groups, with no significant differences observed between them [ ]. A single-arm phase II study investigating the combination of docetaxel and oral curcumin in patients with progressing, chemotherapy-naïve mCRPC demonstrated a higher response rate compared to historical controls treated with docetaxel alone. The combination was well tolerated, with no adverse events attributed to curcumin [ ]. A randomized, controlled trial is needed to definitively establish curcumin’s contribution to the efficacy of the combination.
Three ongoing trials are evaluating the use of curcumin in managing patients with early-stage PCa ( Table 1 ). Turmeric has been associated with liver injury and must be used with caution, especially with the use of black pepper and in patients carrying the HLA-B*35:01 allele, which are thought to increase the risk of turmeric associated liver injury [ ].
3.2
Lycopene
Lycopene is a powerful lipid-soluble, red pigment antioxidant that corresponds to the class of phytochemicals called carotenoids. It is found in high concentrations in tomatoes, tomato-based products, red and pink grapefruits, papaya, pink guava, and watermelon. The bioavailability of lycopene increases after thermal treatment and when consumed with fat or oils. Tissue distribution of lycopene varies, with the highest accumulation in the prostate, testes, adrenal glands, and liver [ ].
Lycopene protects against oxidative damage by increasing the expression of detoxification proteins, including epoxide hydrolase-1, superoxide dismutase-1, catalase, and transferrin [ ]. It increases phase II protective enzymes and downregulates proteins involved in ROS generation [ ]. Lycopene protects against DNA-based oxidative damage, which leads to a decrease in 8-hydroxy-2′deoxyguanosine (8-OHdG) levels, a specific marker for oxidative DNA damage [ ]. At the same time, lycopene at higher than physiological concentrations may result in pro-oxidative effects [ , ]. Lycopene blocks the G1/S phase of the cell cycle with a decrease in cyclin D1 and an increase in the CDK inhibitors: p53, p21Waf1/Cip1, and p27 [ ]. Lycopene increases apoptosis, inhibits cell viability, upregulates p53 and BAX transcript expression, and downregulates Bcl-2 expression in PCa cells [ ]. In LNCaP cells, lycopene decreases the expression of Bcl-2 and Bcl-xL and increases that of BAX [ ]. In PC3 cells, the antiproliferative effect of lycopene results in upregulation of IGFBP-3 expression and downregulation of IGF-1-induced IGF-1R expression [ ]. Lycopene inhibits cell proliferation and induces apoptosis in PC3 cells via the upregulation of miR-let-7f-1 and downregulation of AKT2 expression [ ]. Lycopene inhibits AR gene element expression, leading to the reduction of PSA velocity [ ]. It exhibits antiangiogenic activity through the decrease in VEGF levels [ ]. Lycopene decreases α2β1, αvβ3, and αvβ5 integrins, which may affect PCa cell invasion and migration [ ].
Several studies investigating the relationship between lycopene and PCa risk have yielded mixed results. While most studies demonstrated that higher dietary and circulating lycopene concentrations are associated with lower PCa risk, others reported no effect [ , ]. Similarly, there have been mixed results in the use of lycopene in PCa treatment. For instance, a 3-week lycopene-rich tomato intervention in patients with localized PCa prior to prostatectomy or radiotherapy showed no significant differences in PSA levels between the treatment and control groups. However, a post-hoc analysis revealed that patients with intermediate-risk PCa experienced a significant reduction in PSA levels following lycopene intervention compared to the control group. This suggests that the effect of lycopene may vary depending on the aggressiveness of the disease [ ]. A phase II trial investigating the effect of lycopene on patients with localized PCa prior to radical prostatectomy suggested that lycopene supplementation can decrease PCa growth [ ]. Two trials, one in patients with localized PCa and the other in those with biochemically recurrent PCa (BCRPC), investigated escalating doses of lycopene supplementation on PSA response. No PSA response was observed, despite an increase in plasma lycopene levels from baseline [ , ]. Similarly, a trial with patients with BCRPC receiving lycopene daily yielded no PSA response, though there was a stabilization in PSA levels for a minimum of three months in 95% of patients [ ]. Among patients with progressive mCRPC receiving daily lycopene monotherapy for three months, 35% had a PSA response and 50% had stable PSA with improved ECOG performance status, reduced bone pain, and less urinary tract symptoms [ ]. Similar results were obtained in a study comparing orchidectomy versus orchidectomy-lycopene combination in the treatment of metastatic hormone-sensitive PCa. The combination group resulted in a more durable decrease in PSA levels, reduced bone pain, improved urinary tract symptoms, and longer overall survival compared to the orchidectomy group [ ]. A PSA50 response was not achieved in a phase II pilot study that enrolled patients with progressive CRPC receiving daily lycopene supplementation for 6 months [ ]. A phase II study that examined the combination of docetaxel and lycopene in chemotherapy and biological therapy-naive patients with mCRPC had a PSA response rate of 76.9% and a median overall survival of 35.1 months, efficacy that compared favorably with previously reported studies. However, the study was limited by a small sample size and lack of a control group [ ].
Lycopene has shown promising therapeutic effects in treating PCa and in alleviating cancer-related symptoms. However, its efficacy needs to be validated in larger, well-controlled clinical trials.
3.3
Plumbagin
Plumbagin (5-hydroxy-2-methyl-1,4-naphthoquinone) is a naturally occurring naphthoquinone derived from the roots of the medicinal plant Plumbago zeylanica L . (chitrak). It is also found in Juglans regia (English walnut), Juglans cinerea (butternut and white walnut), and Juglans nigra (blacknut) [ ].
Plumbagin induces apoptosis [ ], inhibits angiogenesis [ ], and decreases AR levels in PCa cells [ ]. It has been shown to significantly enhance the efficacy of ADT and CYP17A1 inhibitors in PCa, leading to increased survival in mice compared to ADT alone. However, when combined with AR antagonists such as bicalutamide and enzalutamide, no additional benefit was observed [ ].
The first-in-human study of PCUR-101, a synthetic form of plumbagin, was conducted as a phase I dose-escalation trial in patients with mCRPC. Patients received increasing doses of oral PCUR-101 in addition to ADT. Due to limited activity, further clinical development of this compound was not pursued [ ].
3.4
Green tea polyphenols (catechins)
Green tea is produced by steaming and drying the fresh leaves from the Camellia sinensis plant. The main antioxidant agents in green tea are catechins, which are polyphenols responsible for the distinctive bitter taste. The four major catechins present in green tea leaves are epigallocatechin-3-gallate (EGCG), epicatechin gallate (ECG), epigallocatechin (EGC), and epicatechin (EC). Among these, EGCG is the most studied and most potent bioactive compound that targets multiple molecular pathways involved in PCa development [ ].
EGCG suppresses tumor growth and cell proliferation of both androgen-sensitive and androgen-independent PCa cells in vitro and in vivo [ ]. It decreases the levels of phosphatidylinositol-3-kinase (PI3K) and phospho-Akt, and increases extracellular signal-regulated kinase (Erk) 1/2 [ ]. EGCG reduces NF-kB transcriptional activity, leading to the decrease in the antiapoptotic protein Bcl-2 levels. It also stabilizes p53 increasing its transcriptional activity, which leads to activation of its downstream targets p21/Waf1 and BAX. The change in the BAX/Bcl-2 ratio favors apoptosis [ ]. EGCG treatment in PCa cells, with or without TP53 mutations, results in dose- and time-dependent upregulation of CDK inhibitors, specifically p21/Waf1, p27/Kip1, p16/INK4a, and p18/INK4c, and downregulation of cyclin D1, cyclin E, CDK2, CDK4, and CDK6, resulting in G0/G1 phase cell cycle arrest [ ]. EGCG increases binding of cyclin D1 toward p21/Waf1 and p27/Kip1 and decreases binding of cyclin E toward CDK2 [ ]. EGCG represses the transcription of the AR gene and downregulates the IGF-1 signaling pathway, thereby slowing the progression of PCa [ , ].
Clinical trials examining the use of green tea or its extracts as a treatment or adjunct therapy for PCa have shown mixed results. Administration of polyphenon E (800mg EGCG) for three to six weeks in patients with PCa prior to prostatectomy showed low prostate tissue bioavailability. There was a decrease in the levels of PSA and IGF-1 and in markers of oxidative DNA damage, but none were statistically significant [ ]. In an open-label, phase II trial, patients with PCa were randomized to six cups daily of brewed green tea, black tea, or water before prostatectomy, which led to a small but significant decrease in PSA levels in the green tea group [ ]. In another phase II trial, patients with progressive CRPC consumed 6 grams of green tea daily for 4 months. Only 1 of 42 patients achieved a PSA50 response, but it was not sustained beyond two months [ ]. A small, single-arm study investigating 250mg of green tea capsules twice daily for up to 5 months in patients with CRPC resulted in no PSA50 responses. Of 19 patients enrolled, only 15 completed the minimum two months of therapy and all were found to have progressive disease within 1 to 5 months of therapy [ ].
Green tea, particularly its catechins such as EGCG, shows potential for PCa prevention and treatment due to its antioxidant, anti-inflammatory, and anticancer properties. However, evidence remains inconclusive, and more research is needed to clarify its benefits and risks. While generally safe in moderate amounts, high doses of green tea extract have been linked to liver toxicity in some cases [ , ]. Therefore, its use as a therapeutic agent should be approached with caution and under medical supervision.
3.5
Soy isoflavones
The phytoestrogen isoflavone, a diphenolic compound, belongs to the flavonoid subgroup. The main sources of isoflavones are legumes from the Fabaceae family, with soybeans being the most common dietary source of daidzein, genistein, and glycitein [ ]. Daidzein undergoes biotransformation by the gut microbiota into equol, which has greater stability, bioavailability, binding affinity to estrogen receptors, and antioxidant activity [ ]. Other sources of isoflavones include red clover (Trifolium pratense) and kudzu root (Radix Pueraria) [ ].
Soy isoflavones share structural similarities with 17β-estradiol, enabling them to bind to both estrogen receptors alpha (ERα) and beta (ERβ). Genistein, a key isoflavone, has an affinity for ERβ that is 20–30 times higher than for ERα [ , ]. The distribution of these receptors varies across tissues; ERα is more abundant in the uterus and mammary glands, while ERβ is more prevalent in the ovary and prostate tissues [ , ]. Physiological concentrations of genistein downregulate AR mRNA and protein expression, and thereby PSA secretion, through ER-β activation [ ]. Genistein inhibits PCa cell growth in culture independent of androgen sensitivity [ ]. In PCa cells, genistein induces a G2/M cell cycle arrest and apoptosis, with downregulation of cyclin B and upregulation of p21/Waf1 growth‐inhibitory protein [ ]. In LNCaP cells, physiologic concentrations of genistein induce a G1 cell cycle arrest and upregulation of p27/Kip1 and p21/Waf1 mRNA and protein expression, leading to potent antiproliferative effects [ ]. Genistein also downregulates PSA mRNA expression [ ]. It inhibits IGF-1-stimulated cell growth and phosphorylation of IGF-1R and the phosphorylation of its downstream targets Src, Akt, and glycogen synthase kinase-3β (GSk-3β) [ ]. Isoflavones inhibit Akt and FOXO3a phosphorylation, downregulate Src signaling, and upregulate GSk-3β expression, which leads to down-regulation of AR and its target gene PSA . This regulation of the Akt/FOXO3a/GSK-3β/AR signaling network leads to the inhibition of cell proliferation and induction of apoptosis [ ]. Genistein inhibits prostate tumor angiogenesis through suppression of VEGF expression [ ].
PCa incidence is significantly higher in Western populations compared to Asian populations, where soy foods rich in isoflavones, such as genistein and daidzein, are widely consumed. Japanese men, for example, have serum isoflavone levels that are 10–100 times higher than those of European men [ ]. A systematic review and meta-analysis by Applegate et al. concluded that higher soy consumption significantly reduces the risk of PCa [ ]. Given this potential benefit, soy is being explored for its therapeutic potential in PCa treatment. A study with oral genistein-rich extract as a sole treatment for six months did not achieve a PSA50 response in 51 of 52 patients with PCa experiencing biochemical failure. However, a stabilization or decline in PSA levels occurred in 8 of 13 patients in the active surveillance group [ ]. A phase II trial investigated the supplementation of 141mg isoflavone daily for 12 months in patients with BCRPC and found a decline of the PSA slope [ ]. Another phase II trial in patients with localized PCa on 30mg of synthetic genistein daily for 3–6 weeks prior to prostatectomy showed a 7.8% PSA decrease in the genistein group and 4.4% PSA increase in the placebo group ( P = 0.051) [ ]. In a double-blind, randomized trial in patients with localized PCa on active surveillance, daily consumption of an aglycone isoflavone supplement (450mg genistein, 300mg daidzein, and other isoflavones) for 6 months showed a significant increase in serum concentrations of genistein and daidzein, but no reduction in PSA levels [ ]. Patients with localized PCa receiving a short course of isoflavones prior to prostatectomy showed no significant changes in PSA compared to the control group [ , ]. Similarly, patients with BCRPC after prostatectomy supplemented with 23–26mg of genistein and 40–43mg of total isoflavones for six to eight months had no difference in PSA doubling time (PSADT) compared to the placebo group [ ]. A pilot study investigating the administration of 200mg soy isoflavone daily for 6 months in patients with localized PCa receiving external beam radiation therapy showed a decrease in radiation-induced adverse effects on bladder, bowel, and sexual function at 3 and 6 months in the soy isoflavone group compared to the placebo group [ ]. Similarly, another pilot study evaluating the effects of 20g soy protein containing 160mg total isoflavones for 12 weeks on the side effects of ADT in patients with PCa showed no significant improvement in vasomotor symptoms, cognition, or other quality-of-life measures in the isoflavone group compared to the placebo group [ ].
Soy isoflavones do not show a promising therapeutic effect on PSA reduction in patients with BCRPC or localized PCa. However, enhancing the biological activity and potential efficacy of soy isoflavones could be achieved through optimizing their biotransformation into equol by the gut microbiota. Factors such as dietary habits, including higher soy consumption in Asian and vegetarian diets [ ], as well as prebiotics and probiotics [ , ], influence gut microbiota composition and equol production. In addition, given the antimetastatic properties of soy isoflavones and the lack of available clinical trials in advanced PCa, clinical trials in this space should be considered [ ].
3.6
Pomegranate
Pomegranate (Punica granatum) is a significant source of two types of polyphenolic compounds: anthocyanins and hydrolyzable tannins. Anthocyanins, including delphinidin, cyanidin, and pelargonidin, are responsible for the red color of the fruit and juice. Hydrolyzable tannins, including punicalin, pedunculagin, punicalagin, gallagic and ellagic acid esters of glucose, account for 92% of the fruit’s antioxidant activity. In pomegranate juice, the amount of soluble polyphenols ranges from 0.2% to 1% [ ].
In androgen-dependent and androgen-independent PCa cells, treatment with pomegranate polyphenols arrests proliferation and stimulates apoptosis through multiple mechanisms and inhibits gene expression of enzymes involved in androgen synthesis and AR signaling [ ]. Pomegranate extract upregulates p21/Waf1 and p27/Kip1 expression, leading to G1 phase cell cycle arrest and apoptosis [ ]. It also upregulates BAX and downregulates Bcl-2 expression, altering the BAX to Bcl-2 ratio to favor apoptosis [ ]. Pomegranate extract inhibits the IGF-1/Akt/mTOR pathway, leading to inhibition of cell survival and growth [ ]. In vivo, oral pomegranate extract significantly reduces tumor growth, with an associated significant decrease in PSA levels in athymic nude mice implanted with androgen-sensitive CWR22Rv1 cells [ ]. It inhibits NF-κB activity in PCa models both in vitro and in vivo [ ]. In an LAPC4 murine xenograft model, pomegranate extract delayed the development of androgen independence by inhibiting proliferation and inducing apoptosis [ ]. The TRAMP mice supplemented with pomegranate extract exhibited significant inhibition of PCa and metastasis development as well as a doubling in overall survival time [ ].
A single-arm, phase II trial in patients with BCRPC assessed the effects of daily consumption of 8 ounces of pomegranate juice until disease progression. The study found a significant increase in PSADT, from a baseline mean of 15 months to a mean of 60 months after treatment. Additionally, there was a 60% reduction in the median PSA slope, decreasing from 0.06 to 0.024 [ , ]. Similarly, the impact of 8 ounces of pomegranate liquid extract on PSADT was evaluated in patients with BCRPC through a double-blind, placebo-controlled trial. Although both the treatment and placebo groups showed a prolongation of PSADT, the difference was not statistically significant. However, a preplanned subset analysis, focused on patients with the manganese superoxide dismutase (MnSOD) alanine/alanine (AA) variant, revealed that those treated with pomegranate extract experienced a statistically significant increase in PSADT from 13.6 to 25.6 months, compared to an increase from 10.9 to 12.7 months in the control group [ ]. In a double-blind phase II trial, patients with BCRPC were randomized to receive either 1g or 3g of pomegranate extract (POMx) daily for up to 18 months. The overall median PSADT increased from 11.9 months at baseline to 18.5 months after treatment. No significant difference was found between the two dosage groups, with an increase in median PSADT from 11.9 to 18.8 months in the low-dose group and from 12.2 to 17.5 months in the high-dose group [ ]. To study if pomegranate juice could be used as an adjunct intervention in patients with advanced PCa, a double-blind, randomized, placebo-controlled phase IIb trial was conducted, with most patients having CRPC. The study found no significant differences in PSA levels between those receiving 500ml of pomegranate juice daily for 4 weeks and those receiving a placebo [ ]. POMx was also evaluated in the neoadjuvant setting for patients with localized PCa prior to radical prostatectomy. Patients were randomized to receive either 2 tablets of POMx, with each capsule containing 1000mg of POMx powder (including 600 mg of polyphenol from extract), or placebo daily for up to 4 weeks. The trial found a 16% reduction in prostatic 8-OHdG, a marker of oxidative stress, in the POMx group compared to the control group, although this reduction was not statistically significant [ ].
While pomegranate extract showed limited efficacy in patients with PCa, a subset of patients with the MnSOD AA genotype demonstrated promising results. Further studies are needed to validate the AA- SOD2 genotype as a genetic biomarker to predict which patients are likely to benefit from pomegranate-based supplements.
3.7
Resveratrol
Resveratrol (3,4′,5-trihydroxy-stilbene) is a plant-derived polyphenolic phytoalexin synthesized in response to environmental stressors. It is present in cis and trans stereoisomeric forms, with trans being the predominant and biologically active form. Mainly concentrated in grape skins and red wine, resveratrol can also be found in raspberries, blueberries, pines, and peanuts [ ].
Resveratrol treatment suppresses androgen-responsive genes, including PSA , human glandular kallikrein-2, AR coactivator ARA70 , and the CDK inhibitor p21 , at both protein and mRNA levels, as a result of the decrease in the AR protein levels [ ]. The impact of resveratrol on AR-dependent gene activity is concentration-dependent, with low concentrations enhancing its expression and higher concentrations repressing it [ ]. In human adrenocortical cells, resveratrol inhibits steroidogenesis by decreasing CYP17 and CYP21 enzyme expression and activity [ ]. Resveratrol inhibits transcriptional activity of ARV7, a splice isoform of AR correlated with the CRPC phenotype [ ]. Resveratrol, alone or in combination with docetaxel, upregulates p53 expression, leading to increased expression of the CDK inhibitors p21/Waf1/Cip1 and p27/Kip1 and decreased expression of the cyclin D1/CDK4 complex, therefore blocking cell cycle progression from the G1 to S phase [ ]. Cell cycle arrest at the G2/M checkpoint is prompted by resveratrol alone or with docetaxel, which is associated with the downregulation of cyclin B1 and CDK1 expression [ ]. Resveratrol promotes apoptosis through the upregulation of the pro-apoptotic proteins, including BAX, BID, and BAK, and downregulation of the anti-apoptotic proteins, including Bcl-2, Mcl-1, and Bcl-xL [ ]. Through AR inhibition, resveratrol stimulates phosphatase and tensin homolog (PTEN) expression, which negatively regulates the PI3K/AKT pathway [ ].
Clinical trials investigating the anticancer effects of resveratrol are limited. A phase I study evaluated increasing doses (500 to 4000mg) of MuscadinePlus (MPX), pulverized muscadine grape skin containing ellagic acid, quercetin, and resveratrol in patients with BCRPC. MPX prolonged PSADT by 5.3 months, but these results were not significant [ ]. This trial was followed by a phase II, randomized, placebo-controlled trial, which found that MPX did not significantly prolong PSADT compared to placebo. However, a notable exception was observed in patients with the SOD2 Ala/Ala genotype, where MPX significantly increased PSADT [ ]. To further investigate, a phase III, randomized, placebo-controlled trial assessed the efficacy of MPX in patients with BCRPC and the SOD2 Ala/Ala genotype. This study found no significant difference in PSA slope or PSADT between the MPX and placebo group [ ].
MPX treatment, which contains resveratrol, did not have a significant effect on PSA reduction in patients with BCR. It is not recommended as a treatment alternative to ADT in patients with BCR.
4
Animal-derived supplements and products
4.1
Omega-3 fatty acids
Omega-3 fatty acids are essential polyunsaturated fats that play a critical role in maintaining overall health. They are particularly well-known for their benefits related to heart health, brain function, and inflammation. In recent years, omega-3 fatty acids have also been studied for their potential impact on cancer, including PCa. Fish is the primary source of omega-3 fatty acids, specifically eicosapentaenoic acid (EPA) and docosahexaenoic acid (DHA) [ ].
Omega-3 fatty acids inhibit the growth and development of PCa through multiple mechanisms. DHA induces apoptosis and autophagy through mitochondrial ROS-mediated Akt-mTOR signaling in TP53 mutated PCa cells [ ]. DHA and EPA prevent the progression of PCa to an androgen-independent state, which was correlated with a decrease in AR expression and suppression of the Akt/mTOR signaling pathway [ ]. In a C3(1) Tag mouse model, a high omega-3 diet was found to slow prostate tumorigenesis by lowering testosterone and estradiol levels, resulting in a decrease in AR expression and downregulation of the expression of genes in the NF-κB and the antiapoptotic Bcl-2 pathways [ ].
Current studies investigating omega-3 fatty acids in PCa have shown mixed results. The effect of 3g/day of fish oil for three months in patients with low-risk PCa on active surveillance resulted in no change in PSA level compared to the placebo group [ ]. A study of patients with low-risk PCa who underwent first active surveillance repeat prostate biopsy concluded that high levels of long chain omega-3 fatty acids in the prostate tissue were associated with a decreased risk of high-grade PCa [ ]. A prospective dietary study was done of patients with untreated, localized PCa consuming a low-fat, fish oil-supplemented diet for three months. This resulted in a significant elevation in the omega-3/omega-6 fatty acid ratios in plasma and adipose tissue but no significant changes in PSA levels [ ]. A population-based cohort of over 500 Swedish men with localized and advanced PCa followed for 20 years post-diagnosis revealed that high marine omega-3 supplementation may improve disease-specific survival [ ]. In a randomized, placebo-controlled, phase II trial, patients with localized PCa received long-chain omega-3 fatty acid supplementation or placebo daily starting seven weeks prior to radical prostatectomy and continuing for up to one-year post-radical prostatectomy. Long-chain omega-3 fatty acid supplementation showed no improvement in overall quality of life, but it did improve urinary function [ ]. In a similarly designed study, omega-3 supplementation did not improve the psychological symptoms, including depression and anxiety, of patients with PCa who underwent a radical prostatectomy [ ]. A phase II trial investigated patients with PCa receiving either a low-fat diet with 5g/day of fish oil or a control Western diet for 4–6 weeks prior to radical prostatectomy. No significant changes in serum IGF-1 or PSA levels were found between the diets, but there was a reduction in malignant epithelial cell proliferation measured by Ki67 immunostaining [ ]. On the other hand, in a phase II trial, patients with PCa receiving 3g/day of EPA esterified in monoacylglycerides (MAG-EPA) for seven weeks prior to radical prostatectomy did not show a difference in Ki67 expression compared to the placebo group [ ]. The effect of 2.4g/day of EPA for 2 years on PSA was studied in patients with PCa postradical prostatectomy. There was no difference in PSA recurrence rates between the treatment and placebo groups [ ].
Although no therapeutic effect of short-term omega-3 supplementation in PCa was observed, long-term use may still provide benefits.
4.2
Protein intake
The relationship between protein intake and prostate cancer outcomes is a topic of ongoing research and debate. While total protein intake alone may not directly affect prostate cancer outcomes, the type of protein consumed appears to play a significant role. Studies have suggested that diets high in animal protein, particularly red and processed meats, may be linked to an increased risk of prostate cancer and its progression [ ]. This potential risk is attributed to factors such as saturated fat content, heme iron, and carcinogenic compounds produced during high-temperature cooking methods like grilling or barbecuing [ , ].
Conversely, plant-based proteins, found in foods such as legumes, nuts, seeds, and whole grains, have been associated with a reduced risk of prostate cancer progression [ ]. Dietary patterns such as the Mediterranean diet, which emphasize plant-based foods and healthy fats, have shown promise in improving prostate cancer outcomes and overall health [ , ]. Plant-based proteins provide additional health benefits as they are rich in fiber, antioxidants, and phytochemicals, which may contribute to their protective effects against cancer.
Although more research is needed to establish definitive conclusions, current evidence supports recommending dietary modifications to patients. Red and processed meats, such as beef, pork, lamb, bacon, sausages, and deli meats, should be limited to reduce potential risks. Instead, increasing the consumption of plant-based proteins like legumes, beans, lentils, nuts, seeds, and whole grains can offer protective benefits. In addition, incorporating fish rich in omega-3 fatty acids, such as salmon and mackerel, and lean poultry into the diet may serve as healthier protein sources. These dietary adjustments may help reduce the risk of prostate cancer and its progression as well as contribute to improved overall health outcomes.
5
Discussion
Natural products have historically played a significant role in cancer treatment, exemplified by drugs such as paclitaxel, vincristine, vinblastine, and etoposide, all derived from natural sources. However, despite growing interest in natural supplements as potential adjuncts in cancer care, opinions vary widely among healthcare professionals, researchers, and patients. While natural supplements offer potential benefits, their integration into cancer treatment presents numerous challenges and concerns.
This review has highlighted that while many natural supplements have shown promising results in preclinical studies (such as in vitro and animal models), clinical trials in PCa have revealed limited or no efficacy. The discrepancy between preclinical and clinical outcomes can be attributed to several factors. Preclinical studies use simplified models that do not account for the complexities of the human body, including metabolism, immune responses, and interactions with other cells. In addition, in vitro and animal studies frequently use higher doses of natural supplements than would be safe or feasible in humans. Moreover, the poor bioavailability of many supplements means that only a small fraction of the ingested substance reaches target tissues in humans, further limiting their effectiveness in clinical trials. Delivery methods used in preclinical studies, such as IV administration, may be impractical or undesirable in human applications, leading to different outcomes. Human cancers are more heterogeneous than the cancer cell lines or animal models used in preclinical research, resulting in variable responses to the same supplement among patients. Preclinical studies also often focus on short-term effects, while human cancers progress over the long term.
Recent advances in drug delivery, such as nanoparticle formulations or chemical modifications of natural products, have substantially improved drug bioavailability [ ]. Using more sophisticated models such as patient-derived xenografts (PDX) and organoids allows for more accurate testing and optimization of natural products in cancer treatment by reflecting individual tumor biology, uncovering mechanisms of action, and personalizing therapeutic strategies. Genetic variations can affect the metabolism, target-specific responses, immune modulation, microbiome interactions, and distribution of natural products, impacting their effectiveness in cancer treatment. Personalized approaches should be employed, including genetic profiling, tailored dosing, and individualized treatment plans. Clinical trials must assess the extended effects and safety of a supplement, requiring longer follow-up periods to evaluate outcomes such as cancer progression, survival rates, and recurrence.
Another significant challenge is that only a few natural supplements have undergone rigorous clinical trials that meet the gold standards of medical research. This gap highlights the need for more high-quality, carefully designed, and adequately powered studies to validate the efficacy and safety of these supplements in cancer treatment. The clinical trials discussed in this review were often limited by small sample sizes, lack of control groups, insufficient study duration, and reliance on PSA as the primary biomarker. To minimize bias and ensure reliable results, clinical trials should be placebo-controlled and double-blinded to help distinguish true effects from placebo effects. Incorporating genomic and molecular profiling into clinical trial design can help identify patients who are more likely to benefit from a specific natural supplement. Identifying and validating biomarkers such as 8-OHdG, reduced glutathione (GSH), and malondialdehyde (MDA) [ ], can help predict a patient’s response to a natural supplement and lead to more personalized treatment approaches.
Marketing for natural supplements often includes exaggerated or unproven claims, which can mislead patients. It is essential that patients rely on scientific evidence rather than anecdotal reports or marketing materials when making decisions about their treatment. A critical concern with natural supplements is the lack of standardization in potency, purity, and quality. Unlike pharmaceutical drugs, supplements are not always rigorously regulated, leading to variability in their contents. While often perceived as safe, some natural supplements can be toxic, especially at high doses. Excessive intake of certain herbs or minerals can result in liver damage, kidney problems, or other health issues [ , ]. For example, turmeric and green tea extract have been associated with liver toxicity [ , , ]. Another risk involves the potential for natural supplements to interact with conventional cancer treatments and daily medications, potentially reducing their effectiveness or causing harmful side effects. For instance, curcumin has been shown to inhibit drug-metabolizing enzymes, such as CYP3A4, and ABCB1 and ABCG2 transporters [ ]. In addition, some patients may delay or forgo conventional cancer treatments in favor of natural supplements, potentially leading to worse outcomes.
Despite these challenges, the continued relevance of natural compounds in cancer research cannot be overlooked. Natural supplements can impact cancer through various mechanisms, both directly by targeting cancer cells and indirectly by supporting overall health. Key mechanisms include antioxidant effects that reduce oxidative stress (e.g., curcumin, vitamin C, lycopene, pomegranate), anti-inflammatory effects that lower cancer risk (e.g., green tea, omega-3, curcumin), and regulation of the cell cycle to prevent cancer cell proliferation (e.g., vitamin D, vitamin E, selenium, resveratrol). Natural supplements can also induce apoptosis in cancer cells (e.g., vitamin E, selenium, soy isoflavones), inhibit tumor angiogenesis (e.g., plumbagin, curcumin, vitamin E), and modulate critical signaling pathways for cell survival (e.g., pomegranate, vitamin C, resveratrol), making them promising candidates for cancer prevention and treatment ( Table 3 ). Combining natural products with 1 another or with standard-of-care medications has the potential to produce synergistic effects. Experts advocate for an integrative approach to cancer treatment, where natural supplements are used alongside conventional therapies under healthcare supervision. This approach ensures that supplements complement, rather than replace, standard treatments such as chemotherapy, radiation, and surgery, potentially enhancing their effectiveness. Some supplements could also help alleviate treatment side effects, improving patients’ quality of life.
