Over the past several decades, clinical trials have demonstrated improved disease-related outcomes in the definitive treatment of anal cancer. Although treatment with radiation and concurrent chemotherapy results in high rates of cure, significant acute and late toxicities are seen. This review focuses on the evolution of treatment-related toxicity for anal cancer. Management of these adverse effects is reviewed, as are future directions in anal cancer treatment and their impact on toxicity.
Key points
- •
Definitive chemoradiation (CRT) regimens in the treatment of anal cancer have evolved over successive clinical trials.
- •
Although cure rates are high with these regimens, they result in hematologic, dermatologic, gastrointestinal (GI), and genitourinary (GU) toxicities.
- •
Alternative strategies, including novel radiotherapy approaches as well as novel chemotherapeutics, are aimed at reducing treatment-related toxicity without having a negative impact on disease-related outcomes.
- •
Toxicity management often requires algorithmic and multidisciplinary approaches; contemporary studies are focusing on long-term quality-of-life sequelae following anal cancer treatment.
Introduction
Anal cancer is an uncommon malignancy whose incidence has been rising over the past several decades. In 2016, it is expected that more than 8000 new cases of anal cancer will be diagnosed in the United States, resulting in more than 1000 deaths. Since the 1970s, curative treatment of nonmetastatic anal cancer has increasingly centered on definitive RT, which now is established as the primary treatment. Although anal cancer is largely curable with CRT, these treatments are associated with a substantial toxicity profile. Many of the large randomized clinical trials (RCTs) for anal cancer have focused on mitigating toxicity without compromising outcomes. This review focuses on the evolution of treatment-related toxicity for anal cancer with the progression of RCTs over the past several decades. As the standard of care has changed with the results of these RCTs, so too has the toxicity profile for anal cancer treatment. Management of these adverse effects is reviewed as are future directions in the treatment of anal cancer and their impact on toxicity.
Introduction
Anal cancer is an uncommon malignancy whose incidence has been rising over the past several decades. In 2016, it is expected that more than 8000 new cases of anal cancer will be diagnosed in the United States, resulting in more than 1000 deaths. Since the 1970s, curative treatment of nonmetastatic anal cancer has increasingly centered on definitive RT, which now is established as the primary treatment. Although anal cancer is largely curable with CRT, these treatments are associated with a substantial toxicity profile. Many of the large randomized clinical trials (RCTs) for anal cancer have focused on mitigating toxicity without compromising outcomes. This review focuses on the evolution of treatment-related toxicity for anal cancer with the progression of RCTs over the past several decades. As the standard of care has changed with the results of these RCTs, so too has the toxicity profile for anal cancer treatment. Management of these adverse effects is reviewed as are future directions in the treatment of anal cancer and their impact on toxicity.
Toxicity during the surgical era
Prior to the advent and acceptance of definitive nonsurgical treatment options for anal carcinoma, abdominoperineal resection (APR) represented the standard of care. From an outcomes perspective, definitive APR alone resulted in recurrence rates of approximately 40% in patients treated with curative-intent. Similarly, 5-year overall survival (OS) rates with APR alone averaged 62%, ranging between 51% and 71% in most studies. In addition to these limitations, APR entails significant morbidity: as a non–sphincter-preserving procedure (ie, the entire anal sphincter complex is removed), permanent colostomy rate is 100%, along with high rates of urinary/sexual dysfunction, wound morbidity, and additional perioperative morbidity and mortality.
With this in mind, Nigro and colleagues at Wayne State University piloted an effort in the early 1970s to assess the role of neoadjuvant CRT, combining 30 Gy to 35 Gy external-beam radiotherapy (EBRT) with concurrent 5-fluorouracil (5-FU) and mitomycin-C (MMC). In their initial report, these investigators demonstrated pathologic complete response (CR) in 2 patients treated with neoadjuvant CRT followed by APR. The possibility of definitive CRT obviating subsequent APR resulted in the expansion of their efforts, culminating in a report of 45 patients treated with 30 Gy EBRT plus concurrent 5-FU/MMC. Remarkably, post-CRT biopsy specimens demonstrated CR in 84% of patients, with no subsequent recurrence in those with biopsy-proven CR. Of these 45 patients, 11% experienced grade 3 or higher hematologic toxicity. The investigators also reported “low-grade stomatitis” and “moderate diarrhea” as part of the acute toxicity profile of their regimen. With these promising results, definitive surgical management of anal carcinoma ultimately gave way to definitive CRT; subsequent RCTs have therefore focused on the optimal nonsurgical approach for anal cancer.
In the modern era, the role of definitive surgical treatment of previously untreated anal lesions is primarily in the setting of T1N0 well-differentiated anal margin cancer. These lesions, with highly favorable prognoses, may be treated with wide local excision; radiotherapy (RT) (with or without concurrent chemotherapy) is reserved for cases where re-excision (for positive or close margins) is not possible. Given this limited scope for definitive surgery, the remainder of this review focuses on the toxicities associated with definitive CRT regimens for these patients.
First-generation trials—radiotherapy versus chemoradiotherapy
Concurrent with the Nigro protocol, other experiences suggested that RT alone could also achieve promising outcomes. Similarly, the toxicities associated with the Nigro chemotherapeutics (in particular MMC) further spurred the question as to whether RT could be as efficacious as CRT but with fewer adverse effects. Two RCTs were conducted to assess outcomes and toxicity profiles with RT versus CRT as definitive treatment of anal cancer: the United Kingdom Coordinating Committee on Cancer Research (UKCCCR) Anal Cancer Trial (ACT I), and the European Organisation for Research and Treatment of Cancer (EORTC) trial. Both ACT I and EORTC trials used a 45 Gy RT regimen and prescribed similar concurrent 5-FU/MCC chemotherapy regimens for the CRT arms. Both trials involved a 6-week post-treatment break and additional boost RT to partial and complete responders; definitive surgery after the 6-week break was pursued for nonresponding patients. Both the ACT I and EORTC trials demonstrated improved locoregional control (LRC) as well as decreased colostomy rates with CRT ( Table 1 ). At 5 years, the LRC rates for the CRT arm versus the RT arm were 68% versus 43% in ACT I and 68% versus 51% in EORTC, with corresponding 5-year colostomy-free survival (CFS) rates of 47% versus 37% and 72% versus 40%, respectively. In long-term follow-up of the ACT I trial, it was further demonstrated that risk of death due to anal cancer was significantly decreased in the CRT group.
Trial | Comparison | Disease-Related Outcomes | Acute Toxicities | Late Toxicities | ||||||||
---|---|---|---|---|---|---|---|---|---|---|---|---|
Locoregional Control | Overall Survival | Colostomy-free Survival | Hematologic | Skin | Gastrointestinal | Genitourinary | Skin | Gastrointestinal | Genitourinary | Other | ||
ACT I (Arnott et al, 1996; Northover et al, 2010) | RT + 5-FU/MMC vs RT-alone | 68% vs 43%, 5 y | 58% vs 53%, 5 y | 47% vs 37%, 5 y | Leukopenia: 7% vs 0%; Thrombocytopenia: 5% vs 0% | Severe skin toxicity: 17% vs 14% | Severe GI toxicity: 5% vs 2% | Severe GU toxicity: 1% vs 0% | 21% vs 18% | Anorectal: 29% vs 27% | 4% vs 4% | Ulcers/radionecrosis: 8% vs 6% |
EORTC (Bartelink et al, 1997) | RT + 5-FU/MMC vs RT-alone | 68% vs 51%, 5 y | 58% vs 53%, 5y | 72% vs 40%, 5y | NR | Grade 3–4 skin reaction: 60% vs 50% | Grade 3–4 diarrhea: 20% vs 8% | NR | Skin ulceration: 6% vs 4%; severe fibrosis: 6% vs 8% | Anal ulcer: 18% vs 4%; anal fistula: 4% vs 6%; anal perforation: 4% vs 4%; rectal stenosis: 6% vs 4% | NR | NR |
With these results, ACT I and EORTC established CRT with 5-FU/MMC as the standard of care for definitive treatment of anal cancer. However, the outcomes benefits observed with the addition of 5-FU/MMC came with the sequelae of increased treatment-related toxicities, primarily skin, GU, GI , and hematologic toxicities (see Table 1 ). In ACT I, which enrolled more than 5-fold the number of patients as the EORTC trial, total early morbidity was significantly higher for the CRT arm (48% vs 39%). Table 1 provides a breakdown of these toxicities. Notably, hematologic toxicity was exclusively observed in the CRT arm. As later studies would confirm, MMC is the primary driver of these acute hematologic effects. ACT I investigators reported 6 chemotherapy-related deaths in the CRT arm (N = 292), 2 of which occurred within 18 months of treatment. These deaths resulted in dose-reduction protocol changes for the frail and elderly. Also reported in ACT I were 3 RT-related deaths (1 in the RT arm [N = 285] and 2 in the CRT arm [N = 292]). With far smaller sample sizes, the EORTC trial reported nonsignificant but higher rates of severe skin reactions and diarrhea in the CRT arm. The sole treatment death in EORTC was in the CRT arm (N = 51), due to neutropenic sepsis during treatment.
Whereas acute toxicities were generally more prominent in the CRT arms of both trials, the late toxicities were similar across treatment groups. In both the initial ACT I and the 13-year long-term follow-up reports, late morbidity was similar between CRT and RT arms, including cutaneous, GU, and GI toxicities (see Table 1 ). The EORTC data echoed these findings, with the exception of a higher rate of anal ulcer development in the CRT arm (18% vs 4%). The EORTC trial also demonstrated no difference in the severe toxicity-free interval survival between the 2 arms. Collectively, these first-generation trials demonstrated superior disease-related outcomes, including CFS, for CRT versus RT, at the expense of increased acute toxicity.
Second-generation trials—mitomycin and cisplatin
Role of Mitomycin—RTOG 87-04
With the establishment of definitive CRT as standard of care, the component parts of the 5-FU/MMC regimen fell under scrutiny. Given the significant toxicity profile of MMC (thrombocytopenia, leukopenia, pulmonary toxicity, nephrotoxicity, and hemolytic-uremic syndrome), smaller trials had attempted to address the efficacy of 5-FU/RT regimens with and without MMC. These efforts culminated in the Radiation Therapy Oncology Group (RTOG) 87-04 trial. This study randomized patients to 5-FU/RT regimens with or without concurrent MMC. Although the RT (45 Gy) and 5-FU regimens were comparable to the ACT I and EORTC trials, the American RTOG 87-04 trial differed from its European counterparts in that MMC was dosed twice—10 mg/m 2 on days 1 and 29 (vs a single dose of 12 mg/m 2 or 15 mg/m 2 on day 1 in the ACT I and EORTC trials, respectively). These differences in MMC dosing between trials, and their potential impact on outcomes and toxicities, are discussed later. At 4-year follow-up, improved disease-related outcomes with the addition of MMC were seen. As shown in Table 2 , the addition of MMC significantly improved CFS (71% vs 59%), local control (84% vs 66%), and disease-free survival (DFS) (73% vs 51%). Although not statistically significant, 4-year OS was also higher in the MMC group (76% vs 67%). These benefits, however, came at the expense of primarily hematologic MMC-related toxicities. Total acute toxicity rate, defined as grade 4 to 5 toxicities experienced within 90 days of treatment initiation, were significantly higher in the MMC arm (20% vs 7%). Stratifying these acute adverse effects, similar nonhematologic toxicities were observed between the arms (7% vs 4%), with hematologic toxicities significantly higher with the addition of MMC (18% vs 3%) (see Table 2 ). The severity of the neutropenia associated with MMC was reflected in the treatment-related deaths as well. Five treatment-related deaths, all due to neutropenic sepsis, were reported: 4 in the MMC arm (N = 146) and 1 in the non-MMC arm (N = 145). Late toxicities were similar between the 2 arms (5% vs 1%) (see Table 2 ). Despite the hematologic effects of MMC, the significant improvement in disease-related outcomes further supported RT + 5-FU/MMC as the standard of care.
Trial | Comparison | Disease-Related Outcomes | Acute Toxicities | Late Toxicities | ||||||||||
---|---|---|---|---|---|---|---|---|---|---|---|---|---|---|
Locoregional Control | Disease-free Survival | Overall Survival | Colostomy-free Survival | Hematologic | Skin | Gastrointestinal | Genitourinary | Other | Skin | Gastrointestinal | Genitourinary | Other | ||
RTOG 87-04 (Flam et al, 1996) | RT + 5-FU/MMC vs RT + 5-FU | 84% vs 66%, 4 y, (LC) | 73% vs 51%, 4 y | 76% vs 67%, 4 y | 71% vs 59%, 4 y | Grade 4–5: 18% vs 3% | Grade 4–5 nonhematologic toxicity: 7% vs 4% | Grade 4–5 late toxicity: 5% vs 1% | ||||||
RTOG 98-11 (Ajani et al, 2008; Gunderson et al, 2012) | RT + 5-FU/MMC vs Induction 5-FU/CDDP– > RT + 5-FU/CDDP | 80% vs 74%, 5 y | 68% vs 58%, 5 y | 78% vs 71%, 5 y | 72% vs 65%, 5 y | Grade 3–4: 62% vs 42% | Grade 3–4: 49% vs 41% | Grade 3–4: 37% vs 47% | Grade 3–4: 3% vs 3% | NR | Grade 3–4: 4% vs 2% | Grade 3–4: 3% vs 2% | NR | Total grade 3–4 Late toxicity: 13% vs 11% |
ACCORD 03 (Peiffert et al, 2012) | Induction 5-FU/CDDP– > RT + 5-FU/CDDP CRT vs RT + 5-FU/CDDP CRT | 80% vs 81%, 5 y, (LC) | 72% vs 65%, 5 y | 72% vs 65%, 5 y | 77% vs 75%, 5 y | Grade 3–4 (during CRT alone): 19% vs 12% | Grade 3–4 Mucositis (during CRT alone): 3% vs 3% | Grade 3–4 diarrhea (during CRT alone): 9% vs 11% | NR | NR | NR | Grade 3–4 diarrhea: 5%; grade 3–4 anal incontinence: 15%; grade 3–4 ulceration/fistula: 12% (all arms) | NR | Grade 3–4 bleeding: 25%; grade 3–4 anal pain: 12% (all arms) |
ACT II (James et al, 2013) | RT + 5-FU/MMC vs RT + 5-FU/CDDP | NR | 69% vs 69%, 5 y | 79% vs 77%, 5 y | 68% vs 67%, 5 y | Grade 3–4 hematologic toxicity: 26% vs 16%; grade 3–4 leukopenia: 24% vs 12%; grade 3–4 febrile neutropenia: 3% vs 3% | Grade 3–4: 48% vs 47% | Grade 3–4: 16% vs 18% | Grade 3–4: 1% vs 2% | Grade 3–4 pain: 26% vs 29% | NR |
Roles of Cisplatin and Induction Therapy—RTOG 98-11, ACCORD 03, and ACT II
Concurrent with RTOG 87-04, phase II trials sought to determine if an alternative chemotherapeutic agent for MMC (namely cisplatin [CDDP], as used with RT in squamous cell carcinoma of other sites, including head and neck, esophageal, and cervical cancers) could optimize the balance between disease-related outcomes and chemotherapy-related toxicities. These trials provided promising results for both CDDP as a substitute for MMC during CRT and CDDP-based induction chemotherapy prior to CRT. These efforts prompted the remaining second-generation RCTs: RTOG 98-11, the UNICANCER ACCORD 03 trial, and the UKCCCR ACT II trial. Despite the differences in study design and execution, all 3 of these RCTs sought to establish the role of CDDP as an alternative to MMC, as well as induction or maintenance chemotherapy in conjunction with CRT.
RTOG 98-11 randomized patients to RT + 5-FU/MMC versus induction 5-FU/CDDP followed by RT + 5-FU/CDDP. The study was terminated on interim analysis due to futility: the primary objective of improved 5-year DFS in the CDDP arm would not be met. Furthermore, the initially reported results of RTOG 98-11 demonstrated significantly lower 5-year colostomy rates in the MMC arm (10% vs 19%). Final reporting of the data for RTOG 98-11, shown in Table 2 , demonstrated that the MMC arm had significantly better 5-year OS (78% vs 71%) and DFS (68% vs 58%), with trends toward better LRC (80% vs 74%) and CFS (72% vs 65%). With regard to toxicity, the MMC arm experienced significantly higher grade 3 to 4 acute hematologic toxicity compared with the CDDP arm (62% vs 42%) but no difference in grade 3 to 4 acute nonhematologic (73% vs 72%) or grade 3 to 4 late toxicities (13% vs 11%). Only 1 treatment-related fatality was reported, due to neutropenic sepsis, in the CDDP arm (N = 324).
The ACCORD 03 trial evaluated a different approach, examining exclusively CDDP-based regimens and the roles of induction chemotherapy as well as higher-dose RT. This trial was predicated on phase II data supporting the use of induction (neoadjuvant) 5-FU/CDDP chemotherapy followed by CRT with 5-FU/CDDP. The trial was also designed prior to the RTOG 98-11 results, which confirmed superiority of up-front CRT with MMC over induction CDDP-based chemotherapy followed by CDDP-based CRT. ACCORD 03 was designed as a 2 × 2 factorial RCT: all 4 arms received definitive treatment with 5-FU/CDDP concurrent with 45 Gy EBRT. Arms A and B received induction chemotherapy (ICT) with 2 cycles of 5-FU/CDDP prior to definitive CRT (thus delaying CRT by 8 weeks); arms C and D received no ICT. After CRT, patients in arms A and C who responded to treatment received a standard RT boost of 15 Gy, whereas responders in arms B and D received high-dose RT boost of 20 Gy to 25 Gy; nonresponding patients in all arms proceeded to APR. The primary outcome measure of ACCORD 03 was CFS, and neither ICT nor higher-dose RT boost resulted in improved disease-related outcomes. ICT arms showed no improvement in CFS at 5 years (arms AB vs CD, 77% vs 75%) nor did the high-dose RT boost arms (arms BD vs AC, 78% vs 74%). Other disease-related outcomes, including DFS, OS, and LRC, were examined, and no significant differences were noted with either the addition of ICT or the higher-dose RT boost. The investigators did not report toxicities by treatment arm, complicating interpretation of the ACCORD 03 data. The acute toxicity data reported, although not subjected to statistical analysis, compared ICT arms to CRT-alone arms (AB vs CD); these data suggested higher rates of grade 3 to 4 acute hematologic toxicity during CRT after ICT (19% vs 12%) (see Table 2 ). These figures also do not include the 15% of ICT patients who experienced grade 3 to 4 hematologic toxicity during ICT. It was further noted that ICT patients had 2-fold higher rates of chemotherapy-dose reduction related to toxicity during CRT (19% vs 9%). Late toxicities were not reported by treatment arm but rather by all patients pooled (see Table 2 ), with 1 to 3 treatment-related deaths occurring in each of the 4 treatment arms (N = 75–82 in each arm); no further details were provided regarding these events, making toxicity assessment in ACCORD 03 more difficult to interpret compared with concurrent RCTs.
Although ACCORD 03 focused on CDDP-based regimens, the last second-generation RCT, ACT II, evaluated MMC-based regimens as the standard approach. Given that the experimental arm of RTOG 98-11 combined both ICT and CDDP-based CRT, the trial left unanswered questions regarding the relative roles of ICT and CDDP-based CRT on outcomes and toxicity rates. To address these issues, ACT II was designed as a 2 × 2 factorial RCT: 1 randomization assigned patients to MMC-based or CDDP-based CRT, and a second assigned patients to receive adjuvant maintenance chemotherapy with 2 cycles of 5-FU/CDDP versus no maintenance therapy. For the first randomization of MMC- versus CDDP-based CRT, the trial examined CR rates as well as acute toxicities with the 2 regimens (in the absence of subsequent maintenance therapy) as the primary endpoints. CR rates at 26 weeks from treatment initiation were similar for the MMC (91%) and CDDP (90%) CRT regimens. Furthermore, the overall acute toxicity profiles did not favor CDDP over MMC (total acute grade 3–4 toxicity, MMC vs CDDP, 71% vs 72%) (see Table 2 ). Acute hematologic toxicities were higher, however, in the MMC arm (grade 3–4, 26% vs 16%), due mostly to increased rates of neutropenia with MMC (grade 3–4, 24% vs 12%). Equal rates of febrile neutropenia were seen in the 2 arms (3% vs 3%). For the 2 nonmaintenance arms (MMC and CDDP, each with N = 246), each arm had 1 treatment death within 4 weeks of treatment completion, and the MMC arm had 1 death 5 to 8 weeks after treatment completion due to non-neutropenic sepsis. Therefore, the higher hematologic toxicity rates with MMC did not translate into increased rates of neutropenic sepsis; given the challenges of CDDP administration (day-long infusion as opposed to 10-minute MMC delivery, requirements for concurrent intravenous hydration with CDDP, CDDP-related neuropathy risk), the investigators concluded that MMC should remain standard of care within the CRT regimen.
The second ACT II treatment randomization (addition of 2 cycles of 5-FU/CDDP maintenance chemotherapy vs none) did not affect long-term disease-related outcomes. DFS at 3 years was no different with or without maintenance chemotherapy (74% vs 73%, respectively). Long-term disease related outcomes were also no different in the non-maintenance MMC and CDDP arms, respectively, including DFS, OS, and CFS (see Table 2 ). Late-term toxicity was not reported in the trial. Collectively, ACT II demonstrated that maintenance therapy provided no outcomes benefits, and the standard of care for definitive management of anal cancer remains RT + 5-FU/MMC CRT.
Treatment Gap
One important issue raised by many of these trials is the significance of gaps, or delays in treatment. The ACT I, EORTC, and RTOG 87-04 trials all included a scheduled 4-week to 6-week treatment break after the initial 45 Gy, followed by RT boost, with boost dose determined by tumor response to initial treatment. The ACCORD 03 trial similarly included a 3-week treatment gap between initial 45 Gy and boost delivery. In contrast, RTOG 98-11 delivered a boost RT dose immediately following initial CRT for select patients, and ACT II consolidated all RT into a continuous 50.4 Gy regimen. Multiple studies have sought to address what effects these variations in treatment course, including treatment breaks and boost doses, might have on outcomes. One analysis of the ACT I data suggested that the RT boost 6 weeks following the initial 45 Gy RT did not increase local control but rather increased late toxicity, in particular radionecrosis. Another analysis, pooling the RTOG 87-04 and RTOG 98-11 trial data, demonstrated that total treatment duration was associated with higher rates of local failure as well as colostomy failure. Two phase II trials further evaluated this issue. RTOG 92-08 attempted to deliver an escalated RT dose to 59.6 Gy using split course treatment (with a 2-week break during RT). Comparing the patients of RTOG 92-08 to those of RTOG 87-04 (MMC arm), it was noted that the split course of treatment resulted in a lower incidence of grade 3 to 4 acute skin toxicity but markedly higher colostomy rates at 1 year (23% vs 6%) and 2 years (30% vs 7%). Long-term follow-up of RTOG 92-08 determined that split-course patients had low rates of late toxicity but lower DFS and CFS. These conclusions were supported by ECOG 4292, another phase II dose-escalation trial, in which patients received 59.4 Gy either with or without a planned 2-week treatment break during RT. The cohort with the planned treatment break had markedly inferior 5-year OS (58% vs 84%) and progression-free survival (53% vs 85%) compared with the continuous cohort. These data suggest improved disease-related outcomes with no planned treatment break. Along these lines, the absence of break or delay in the ACT II treatment scheme is regarded as one reason for the high CR and DFS rates relative to ACT I. Despite the potential advantages of completing treatment without breaks, prior series have suggested that many patients require a break, and a small minority have to stop treatment entirely, both poor predictors of outcome. Mitigating toxicities that lead to treatment breaks or cessation, therefore, is an important endpoint in optimizing outcomes. To that end, newer radiation techniques, in particular intensity-modulated RT (IMRT), have been implemented in the treatment of anal cancer.
Prospects of intensity-modulated radiotherapy
Existing RCT data for anal cancer, from ACT I through ACT II, rely on older radiation techniques with homogenous radiation intensity in a given treatment field. These techniques, including 2-D (anteroposterior-posteroanterior [AP-PA]) RT or 3-D conformal RT (3D-CRT), deliver relatively high doses to tissues surrounding the treatment target. In contrast, IMRT allows for modulation of radiation intensity with a given field and often relies on a large number of fields for RT delivery (generally 9 or more fields for pelvic malignancies). In IMRT-based planning, strict radiation dose constraints to normal organs are established, doses are prescribed to different target volumes, and computer software inverse planning algorithms are used to design unconventional treatment fields that would not otherwise be possible with standard planning methods. IMRT involves partitioning of a given radiation field into multiple smaller fields, which can occur in the form of dynamic IMRT (in which collimating leaves, or blocks, move across an active radiation field using highly specific leaf sequences) or step-and-shoot IMRT (in which leaves sculpt the field shape while the beam is off). The end result of these approaches is that the intensity of the radiation beam for any one field varies. When these IMRT fields are summed, the cumulative effect is a radiation dose distribution that closely conforms the radiation dose around the tumor or target volumes while significantly reducing the dose to surrounding normal tissues. It was therefore postulated that IMRT might decrease toxicity by decreasing dose to surrounding normal tissues and consequently result in fewer treatment breaks as well as decreased overall treatment time. Several single-arm retrospective (as well as a few prospective) series examined IMRT-based CRT in the definitive treatment of anal cancer, with lower rates of acute toxicities (primarily GI and skin) relative to the MMC arm of RTOG 98-11 as a 3D-CRT reference for comparison ( Table 3 ). Several series reported their institutional experiences comparing patients treated with 3D-CRT and IMRT, with similarly improved GI and skin toxicities (see Table 3 ). As discussed elsewhere, these IMRT series report comparable disease-related outcomes compared with the MMC arm of RTOG 98-11.
Study | Notes | N | Acute Toxicities (Grade 3–4), % | |||
---|---|---|---|---|---|---|
Hematologic | Skin | Gastrointestinal | Genitorurinary | |||
Prospective trials | ||||||
RTOG 98-11 (Ajani et al, 2008; Gunderson et al, 2012) | RT + 5-FU/MMC arm (RT with 3D-CRT for comparison) | 325 | 62 | 49 | 37 | 3 |
RTOG 05-29 (Kachnic et al, 2013) | IMRT + 5-FU/MMC | 52 | 58 | 23 | 21 | 2 |
Single-arm IMRT series | ||||||
Milano et al, 2005 | (Retrospective) | 17 | 53 | 0 | 0 | 0 |
Salama et al, 2007 | (Retrospective) | 53 | 59 | 38 | 15 | 0 |
Pepek et al, 2010 | (Retrospective) | 29 | 24 | 0 | 16 | 3 |
DeFoe et al, 2012 | (Retrospective) | 78 | 13 | 29 | 28 | NR |
Kachnic et al, 2012 | (Retrospective) | 43 | 51 | 10 | 7 | 7 |
Vieillot et al, 2012 | (Retrospective) | 39 | 27 | 42 | 10 | 5 |
Han et al, 2014 | (Prospective) | 58 | 41 | 46 | 9 | 0 |
Janssen et al, 2014 | (Retrospective) | 25 | 19 | 24 | 0 | 0 |
Mitchell et al, 2014 | (Retrospective) | 65 | 3 | 17 | 9 | 2 |
Belgioia et al, 2015 | (Retrospective) | 41 | 5 | 5 | 7 | 0 |
Franco et al, 2015 | (Prospective) | 54 | 17 | 13 | 8 | 2 |
Yates et al, 2015 | (Retrospective) | 42 | 19 | 33 | 14 | NR |
Call et al, 2016 | (Retrospective) | 152 | 41 | 20 | 11 | 0 |
Comparison series | ||||||
Saarilahti et al, 2008 | (Retrospective, 3D-CRT vs IMRT) | 39, 20 (3D-CRT, IMRT) | NR | 82 vs 80 | 31 vs 0 | 35 vs 7 |
Bazan et al, 2011 | (Retrospective, 3D-CRT vs IMRT) | 17, 29 | 29 vs 21 | 41 vs 21 | 29 vs 7 | NR |
Dewas et al, 2012 | (Retrospective, 3D-CRT vs IMRT) | 27, 24 | 4 vs 4 | 35 vs 38 | 4 vs 4 | NR |
Chuong et al, 2013 | (Retrospective, 3D-CRT vs IMRT) | 37, 52 | 38 vs 29 (leukopenia) | 65 vs 12 | 30 vs 10 | 5 vs 0 |
Koerber et al, 2014 | (Retrospective, 3D-CRT vs IMRT) | 37, 68 | NR | 95 vs 63 (grade 2–3) | 68 vs 47 (grade 2–3) | NR |
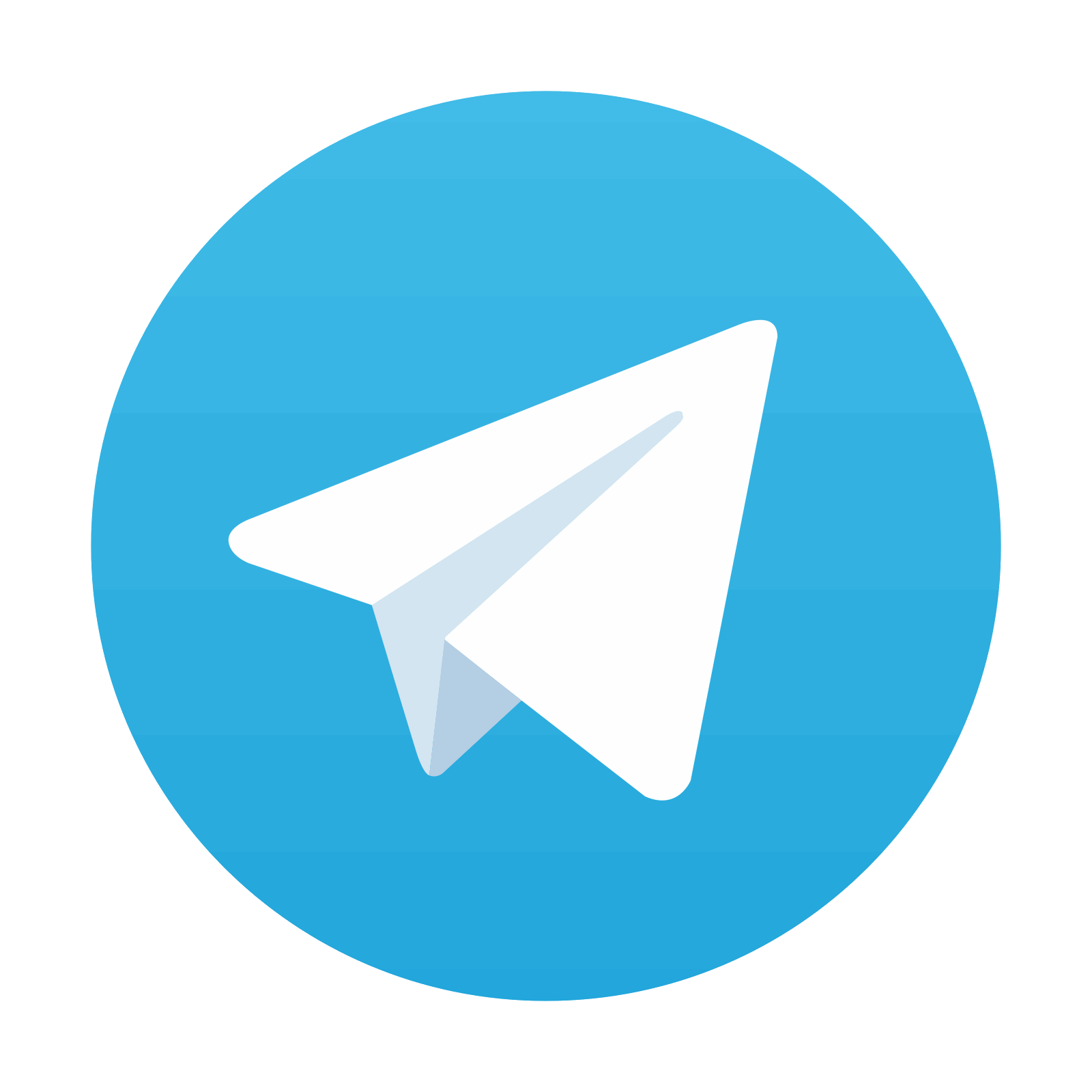
Stay updated, free articles. Join our Telegram channel

Full access? Get Clinical Tree
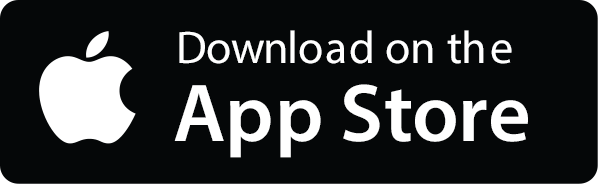
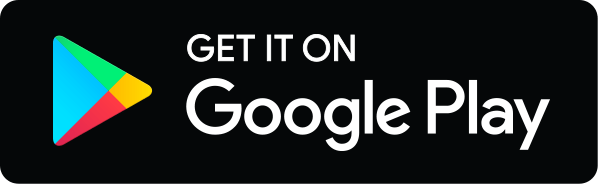
