Characteristic
TheraSphere®
SIR-Sphere®
Isotope
90Y
90Y
Half-Life (h)
64.2
64.2
Particle Size (μm)
20–30
20–60
Range of spheres per vial
1.2–8.0 million
40–80 million
Activity per sphere (Bq)
2500
50
Specific gravity
High
Low
Activities available (GBq)
3, 5, 7, 10, 15, 20
3
Requires handling for dispensing
NO
Yes
Modern delivery route
Transcatheter, intra-arterial (hepatic)
Transcatheter, intra-arterial (hepatic), hepatic ports (rare)
Embolic effect
Mild
Moderate
Indications for use
HCC with appropriately positioned catheter
Colorectal metastases with intrahepatic floxuridine
Special radiation precautions upon discharge
None
Possible urine contamination
TheraSphere® (MDS Nordion, Ottawa, Canada) consists of nonbiodegradable glass microspheres that have a diameter between 20 and 30 microns. It was approved by the FDA in 1999 as a bridge to transplantation [21]; and recently has been approved for use in HCC patients with PVT. Vials of six different activities are available and they differ from each other in the number of spheres per vial e.g., 1.2 million microspheres are present in a vial with an activity of 3 Gigabecquerel (GBq). Each microsphere has an activity of 2,500 Becquerel at the time of calibration. The activity of the vial varies inversely with the time elapsed after calibration and the usable shelf life of a TheraSphere® dose is 12 days from calibration [22].
SIR-Spheres® (Sirtex, Lane Cove, and Australia) consist of biodegradable resin microspheres. The spheres are slightly larger and less dense than TheraSphere® and hence associated with more embolic effect. Only one vial of SIR-Spheres® of 3 GBq is available and contains 40–80 million microspheres measuring from 20 to 60 microns. The use of SIR-Spheres® was approved by the FDA for metastatic colorectal cancer to the liver. Each microsphere has a specific activity of 50 Bq at the time of calibration. The usable shelf-life of the device is 48–72 h.
3 Technical Considerations Before 90Y Microspheres Administration
Radioembolization is the term used for administration of 90Y microspheres. It is defined as the injection of micron-sized embolic particles loaded with a radioisotope by use of percutaneous transarterial techniques. Flow dynamics and tumor hypervascularity allow the microspheres to preferentially travel to the distal arterioles within the tumors, where beta-emissions from the isotope irradiate the tumor. External radiation has played an inconsequential role in liver malignancies due to the radiosensitivity demonstrated by hepatic tissue and consequent development of radiation-induced liver disease (RILD), a clinical syndrome of ascites, anicteric hepatomegaly, and elevation of liver enzymes upon the exposure to doses greater than 35 Gray (Gy) [23, 24]. Besides, the dose given via external radiation is not sufficient to kill cancer cells. Radioembolization helps overcome both of these issues to a great extent. This is an outpatient procedure.
3.1 Vascular Anatomy of the Liver
Liver has dual blood supply i.e., from portal vein and hepatic artery proper. Portal vein which carries venous blood form gastrointestinal tract (GIT) to liver is the predominant source of vascular supply for hepatocytes to carry out metabolic functions. The hepatic artery proper is a branch of common hepatic artery which itself arises from celiac trunk. It divides into right and left branches which supply corresponding lobes. Right hepatic artery also gives off cystic artery that supplies gallbladder. Interestingly, normal hepatocytes are supplied by portal blood, whereas cancer cells dwell on blood supplied by hepatic artery [25–28]. This fact is well exploited by intra-arterial therapies and forms the basis for prioritizing the delivery of cytotoxic therapy to the capillary bed of tumor cells via the arterial route while limiting the exposure to normal hepatocytes. Given this fact, radiation dose up to 150 Gy can be selectively administered to the tumor minimizing the complications of external radiation.
3.2 Pretreatment Evaluation
All patients undergo pretreatment assessment consisting of history, laboratory, and imaging work-up. Diagnostic criteria for HCC included those defined by guidelines [29] and are beyond the scope of this discussion. Pretreatment cross sectional imaging is essential for treatment planning and post treatment response assessment. All patients undergo following essential pretreatment evaluation procedures approximately 1 week before the planned first treatment, and are not usually repeated for any subsequent treatments.
3.2.1 Pretreatment Angiography
Given the propensity for arterial variants and hepatic tumors to exhibit arteriovenous shunting, all patients being evaluated for 90Y must undergo pretreatment mesenteric angiography [21, 26]. This, on one hand, allows for tailoring the treatment plan according to each patient`s individual anatomy and on the other, helps us assess any inadvertent spread of the microspheres to nontarget organs, [30] which can be controlled by prophylactic embolization.
The aortogram is performed to assess the tortuosity and the presence of atherosclerosis in the aorta. The superior mesenteric and celiac trunk angiograms allow interventional radiologists an opportunity to study the vascular anatomy of the liver. The patency of the portal vein and the presence of arterio-portal shunting are also assessed. Prophylactic embolization of the gastroduodenal artery and right gastric artery is recommended as a safe and efficacious mode of minimizing the risks of hepato-enteric flow, particularly if using resin microspheres [26, 31, 32]. Other vessels that may need to be embolized are the falciform, inferior esophageal, left inferior phrenic, accessory left gastric, supraduodenal, and retroduodenal arteries.
Diagnostic angiography is essential to ensure that the blood supply to the tumor(s) has been adequately identified as incomplete identification of the blood supply to the tumor may lead to incomplete targeting and treatment. It also allows for accurate calculations of target volumes [22].
3.2.2 Pulmonary Shunting and Technetium-99m Labeled Macroaggregated Albumin (99mTc-MAA) Scans
One of the angiographic features of HCC is direct arteriovenous shunting bypassing the capillary bed in contrast to metastatic tumors to the liver [33]; therefore, shunting of 90Y microspheres to lungs becomes a concern possibly resulting in radiation pneumonitis [34]. Because the size of 99mTc-MAA particle closely mimics 90Y, it is assumed that distribution of the two will be identical as well and this concept is utilized in assessing splanchnic and pulmonary shunting. It is important to correlate the findings of angiography to the findings of the 99mTc-MAA scan as the proximity of some portions of the GIT to the liver may confuse the findings of these nuclear medicine scans. Lung shunt fraction (LSF) is used to calculate the dose delivered to the lungs and appropriate adjustment for this parameter minimizes the risk of radiation pneumonitis.
3.3 Dose Calculation
3.3.1 Dose Calculation for TheraSphere®
The volume of infused liver in cubic centimeters is calculated using 3-dimensional reconstruction of the target site. This value is used to calculate the mass of infused liver tissue in grams by multiplying it by a factor of 1.03. The activity administered to the target area of the liver (A) in GBq, assuming uniform distribution of microspheres, is calculated using the following formula:
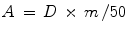
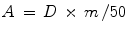
Where D is the dose administered in Gy and m is the mass in kilograms of the tissue to be perfused with microspheres (kg).
Dose delivered to the treated mass also depends on the percent residual activity (R) in the vial after treatment and the LSF which is calculated beforehand using the 99mTc-MAA scan. These factors are accounted for in the following formula,


3.3.2 Dose Calculation for SIR-Spheres®
The dosimetry model for SIR-Spheres® is based on whole liver infusion. The calculated activity in GBq of the whole liver is multiplied by the ratio the target site to the whole liver. Although there are three methods for dosimetry of SIR-Spheres®, only one is recommended. The partition method is seldom used as it is applicable only in special circumstances. The simple empiric method is based on percent tumor involvement of liver and recommends doses of 2 GBq for ≤25 % involvement, 2.5 GBq for 25–50 % involvement, and 3 GBq for >50 % involvement.
The most widely used body surface area (BSA) method is calculated as follows,


Where A is the activity in GBq, BSA is the BSA in meters squared (m2), and percent tumor burden is the percentage of the liver that is involved by tumor. Often, a 20 % dose reduction factor is applied.
4 Clinical Aspects of 90Y Microspheres Administration
4.1 Patient Selection
All patients undergo clinical evaluation which includes history, physical examination, laboratory profile including liver function, and detailed imaging. Patients are selected according to following criteria.
4.1.1 Inclusion Criteria
(a) Confirmed diagnosis of surgically unresectable HCC or intrahepatic cholangiocarcinoma (ICC) or metastatic disease, (b) age >18 years, (c) Eastern Cooperative Oncology Group (ECOG) performance status ≤2, (d) adequate pulmonary function test findings, (e) adequate hematologic parameters (granulocyte count >1.5 × 109/L, platelet count >50 × 109/L), renal function (serum creatinine level <2.0 mg/dL) and liver function (serum bilirubin level <3.0 mg/dL), (f) ability to undergo angiography and selective visceral catheterization, and (g) potentially resectable/transplantable by imaging (but not medical comorbidity criteria) [35, 36].
4.1.2 Exclusion Criteria
(a) Any other liver therapy planned for cancer treatment, (b) uncorrectable flow to the GIT, (c) estimated radiation doses to the lungs greater than 30 Gy in a single administration or 50 Gy in multiple administrations, and (d) significant extrahepatic disease representing imminent life-threatening outcome.
4.2 90Y Radioembolization Procedure
The procedure for administering 90Y should be followed with care and caution. The apparatus for the administration of 90Y is designed to minimize radiation exposure to persons involved in the procedure. The tumor is approached under fluoroscopic guidance and the vial is injected into the vessel feeding the tumor. Tumor distribution guides for the selectivity of the treatment i.e., to one or more lobes/segments as required. A physicist should be present throughout the case to ensure that proper protocols are followed to minimize accidental radiation exposure. There are distinct differences in the infusion techniques for TheraSphere® and SIR-Sphere®.
4.2.1 TheraSphere® Infusion Technique
Infusion should be performed using 3 French systems. The delivery of TheraSphere® is dependent on blood flow through the hepatic vasculature distal to catheter tip so it is necessary to make certain that catheter does not occlude the vessel in which it is placed; otherwise vessel spasm may cause reflux. The rate of infusion should mimic the rate of hepatic arterial flow which can be assessed by visual inspection of a test dose of contrast before TheraSphere® administration [25]. The usual infusion time for TheraSphere® is 3–5 min.
4.2.2 SIR-Spheres® Infusion Technique
Similar to TheraSphere®, most infusions for Sir-Sphere® are performed through 3 French catheter systems. There is no pressure gauge on the SIR-Spheres® delivery kit and hence pressure cannot be monitored; however, the low specific gravity of the microspheres allows the egress of the device via the catheter in a relatively low-pressure state. Given the embolic load of SIR-Spheres®, it is also necessary to make certain that the catheter does not occlude the vessel in which it is placed to prevent reflux. Given the larger number of microspheres and lower specific activity, it is possible for the entire vascular bed to become saturated with microspheres and an embolic state to be reached. For this reason, fluoroscopic guidance is essential during the infusion. The technique of infusion involves the alternating infusion of sterile water and contrast, never allowing direct SIR-Spheres® contact with contrast [28]. Recently, investigators are recommending an infusion time of 30–45 min for SIR-Spheres®.
Both SIR-Sphere® and TheraSphere® infusion procedures are performed on outpatient basis. Patients are discharged on a 2-week prescription of proton pump inhibitors for GI ulcer prophylaxis [22].
4.3 Post-treatment Assessment
Clinical, laboratory and radiological follow-up is essential to monitor response to treatment and identify any toxicity. Regular laboratory follow-up includes the hepatic panel and tumor markers. Cross sectional imaging is performed 1 month post-treatment and then every 3 months to assess response to treatment or progression of disease.
4.4 Response Assessment Following Radioembolization
4.4.1 Imaging Following Radioembolization
CT and MRI are used for response assessment. In addition to conventional cross-sectional imaging such as CT and MRI, positron emission tomography (PET) has a role in assessing response to treatment for secondary liver tumors.
Given the lack of standardization of functional imaging in HCC, anatomical methods are still considered the gold standard for response assessment. In 1979, the World Health Organization (WHO) (bidimensional perpendicular measurements) published guidance on the anatomical assessment of tumor response to therapy [37]. In 2000, the Response Evaluation Criteria in Solid Tumors (RECIST) guidelines (unidimensional measurements) were published, updating the WHO document [38]. European Association for Study of the Liver (EASL) guidelines were published in 2001 and were based on percent change in amount of enhancing tumoral tissue post-treatment [38–40]. The EASL guidelines address the limitations of WHO and RECIST guidelines as they were intended for systemic therapies and there were limitations in applying them for locoregional therapies like radioembolization.
Recent Studies
Riaz et al. in a series of 35 patients who underwent radioembolization correlated post-treatment radiologic findings to pathologic data [41]. They concluded that changes in amount of enhancing tissue and size of a tumor following therapy are predictors of complete pathologic necrosis.
In a recent series of 245 patients treated with locoregional therapies, Riaz et al. investigated the concept of Index lesion and inter-method agreement among RECIST, WHO and EASL guidelines. They concluded that there is high agreement between RECIST and WHO guidelines but low between each of these and EASL. Moreover, primary index lesion which is the dominant lesion can be used to measure response to therapy by applying the above-mentioned guidelines [13].
In another study, Riaz et al. compared various combinations of WHO, EASL and RECIST guidelines and devised various scoring systems based on the combinations of these guidelines. They concluded that EASL × WHO scoring system provides a simple and clinically acceptable method of response assessment and radiological–pathological correlation [42].
Rhee et al. concluded that HCC tumor response using diffusion-weighted imaging changes after Yttrium-90 radioembolization (90Y) preceded anatomic size changes. Conventional anatomic imaging studies are not able to assess tumor response until 6 weeks have elapsed after treatment and functional MRI may have a role in earlier detection of tumor response [43].
4.4.2 Tumor Biomarkers
Riaz et al. investigated the role of alpha fetoprotein (AFP) in locoregional therapies; they concluded that AFP response seen after transarterial locoregional therapy could be used as an ancillary method of assessing tumor response and survival [44].
Although utilized clinically, the role of other tumor markers such as carcinoembryonic agent and CA 19-9 in assessing response following radioembolization of ICC have not been studied.
4.5 Indications for Radioembolization in Primary Liver Malignancies and Recent Studies
4.5.1 Hepatocellular carcinoma (HCC)
Patients within Milan criteria i.e., single lesion less than 5 cm or less than or up to three lesions all less than 3 cm, are eligible for orthotopic liver transplantation (OLT) [16]. Resection is possible only if liver function is well-compensated. The use of surgical options is considered the gold standard for these patients. The limited availability of donor organs for OLT and the dropout of patients due to tumor progression limit the number of patients who initially were within transplant criteria and are able to undergo OLT.
Patients who are outside transplant criteria but do not have malignant PVT or metastatic HCC are also candidates for some targeted therapies. Radioembolization in these patients has been shown to downstage the disease within transplant criteria [12]. This use allows the patients who were initially outside Milan criteria to be eligible for transplant. Recurrence-free and overall survivals after OLT in the downstaged patients are yet to be compared to those of patients who were already within transplant criteria to determine the efficacy of downstaging. This group of patients may also have a survival benefit following treatment even without downstaging.
The use of radioembolization has been shown to limit progression of the disease in HCC. This helps in bridging the patient to OLT as it allows the patient more time to wait for donor organs [45]. Lewandowski et al. compared chemoembolization to radioembolization in their retrospective analysis patients with HCC beyond Milan criteria [12]. Radioembolization was shown to be a better tool than chemoembolization for downstaging the disease to within transplant criteria.
In a Phase 2 study analyzing the long-term outcomes of patients treated with 90Y for HCC, Salem et al. concluded that patients with Child-Pugh A disease, with or without PVT, benefited most from the treatment, patients with Child-Pugh B disease who had PVT had poor outcomes, whereas TTP and overall survival varied by patient stage at baseline [10]. Further results of this study are presented in Table 2.
Table 2
Results from a phase 2 study assessing long-term outcomes of radioembolization for hepatocellular carcinoma by Salem et al. [9]
Variable | Findings | |
---|---|---|
No. of patients | 291 | |
No. of treatments | 526 (mean 1.8/patient) | |
Toxicities | Fatigue (57 %), pain (23 %), nausea/vomiting (20 %), Grade ¾ bilirubin toxicity (19 %) | |
WHO response | Child-Pugh A: 52 % | BCLC A: 46 % |
Child-Pugh B: 39 % | BCLC B: 51 % | |
Child-Pugh C: 0 % | BCLC C: 40 % | |
BCLC D: 0 % | ||
EASL response | Child-Pugh A: 69 %
![]() Stay updated, free articles. Join our Telegram channel![]() Full access? Get Clinical Tree![]() ![]() ![]() |