© Springer International Publishing AG 2017
Doruk Erkan and Michael D. Lockshin (eds.)Antiphospholipid Syndrome10.1007/978-3-319-55442-6_1616. 15th International Congress on Antiphospholipid Antibodies Task Force on Pediatric Antiphospholipid Syndrome Report
Arzu Soybilgic1, Cassyanne L. Aguiar2, M. Patricia Massicotte3, Gili Kenet4, E. Ann Yeh5, Laura Andreoli6, Tadej Avcin7 and Barry L. Myones8
(1)
Pediatric Rheumatology, Children’s Hospital of University of Illinois, Chicago, IL, USA
(2)
Pediatric Rheumatology, Children’s Hospital of The King’s Daughters, Eastern Virginia Medical School, Norfolk, VA, USA
(3)
Department of Pediatrics, University of Alberta, Stollery Children’s Hospital, Edmonton, Alberta, Canada
(4)
Sheba Medical Center at Tel Hashomer, Ramat-Gan, Tel Aviv, Israel
(5)
Division of Neurology, Department of Pediatrics, Hospital for Sick Children, University of Toronto, Toronto, Ontario, Canada
(6)
Department of Clinical and Experimental Sciences, Unit of Rheumatology and Clinical Immunology, Spedali Civili di Brescia, University of Brescia, Brescia, Italy
(7)
Children’s Hospital, University Medical Center Ljubljana; and Faculty of Medicine University of Ljubljana, Department of Allergology, Rheumatology and Clinical Immunology, Ljubljana, Slovenia
(8)
Pediatric Rheumatology, Sugar Land, TX, USA
Keywords
Pediatric antiphospholipid syndromeDevelopmental hemostasisHemostatic testingThrombosis risk assessmentTreatmentClassification criteriaIntroduction
The antiphospholipid syndrome (APS) is an autoimmune multisystem disease characterized by thromboembolic events, pregnancy morbidity, and hematological, dermatological, neurological, and other manifestations in the presence of elevated titers of antiphospholipid antibodies (aPL) [1]. Antiphospholipid syndrome may occur as an isolated clinical entity (primary APS) or in association with other diseases, mainly systemic lupus erythematosus (SLE) . It occasionally occurs with other autoimmune conditions, infections, and malignancies. Pediatric APS due to de novo production of aPL occurs anytime from the neonatal period through childhood and adolescence. Neonatal APS, manifesting with thrombotic events, is a rare complication; it may be associated with maternal aPL, in which case it is attributed to transplacental passage of aPL, or it may be found in newborns whose mothers do not have aPL. The rapid development of multiple organ thromboses and microthromboses, known as catastrophic APS (CAPS) , has been reported in children [2].
In recognition of the unique issues surrounding the diagnosis and treatment of pediatric APS patients, a pediatric APS task force was developed in preparation for the 15th International Congress on aPL (www.apsistanbul2016.org) (North Cyprus, September 2016). This multidisciplinary group consists of pediatric rheumatologists, pediatric neurologists and neurodevelopment specialists, pediatric hematology-coagulation specialists, and neonatal-perinatal medicine specialists. The objectives of this task force were to review the current knowledge on the pathogenesis, clinical and laboratory features, diagnosis, classification, and treatment of the pediatric APS to generate recommendations for future research and to suggest modifications to the APS classification criteria for enhanced applicability to children and neonates. Members of this task force have published two extensive reviews of the literature [3, 4].
A Brief Review of Hemostasis
Hemostasis, the normal reparative process for damaged vasculature, is a three-stage process (vessel damage and platelet response, thrombin generation, and fibrinolysis) with the following participants: cells or cellular remnants (platelets, monocytes, endothelial cells, and microparticles), proteins (hemostatic and fibrinolytic), and vascular endothelium, together known as the cell-based model of hemostasis [5–7].
Inhibitory mechanisms that modulate hemostasis include blood proteins (proteins C and S, antithrombin [AT], α2-macroglobulin, and tissue factor pathway inhibitor [TFPI]); vessel wall proteins (thrombomodulin, endothelial protein C receptor, and TFPI) or chemicals (nitrous oxide [NO], CD39 [ectonucleotidase]); prostacyclin; and proteoglycan-related glycosaminoglycan molecules (chondroitin, dermatan sulfate, or heparin sulfate) [8, 9].
Vessel Damage and Platelet Response (Adhesion, Activation, and Aggregation)
The first stage of hemostasis is initiated following blood vessel damage as a result of vessel severing, for instance, surgery, trauma, or clinical conditions like cancer [7]. Von Willebrand factor (vWF) adheres to the exposed collagen within the damaged vessel wall and attracts platelets through receptor glycoprotein (GP)-Ib-IX-V [10]. This binding is not firm, but it causes platelet GPIIb-IIIa receptor (outside-in signaling) to become active and bind to vWF. Platelets adhere to the area of damage, express negatively charged phospholipids (primarily phosphatidylserine) [11], become activated, and release granule contents. Alpha granules secrete hemostatic factors (factor V [FV], vWF, fibrinogen), angiogenic factors (angiogen, vascular endothelial growth factor [VEGF] ), anti-angiogenic factors (anti-angiostatin, platelet factor 4), growth factors (platelet-derived growth factor [PDGF], basic fibroblast growth factor [bFGF], stromal cell-derived factor 1 [SDF1α]), proteases (matrix metallopeptidase 2 [MMP2], matrix metallopeptidase 9 [MMP9]), necrosis factors (tissue necrosis factor α [TNFα], tissue necrosis factor β [TNFβ]), and other cytokines. Dense granules secrete the small molecules serotonin, adenosine diphosphate (ADP), and polyphosphates [12]. Many of the components promote further platelet activation. Fibrinogen binds to the platelet receptor, GPIIb-IIIa, and, through multiple receptor binding sites, binds activated platelets to form an aggregated mass [13]. Inhibitory mechanisms of platelet aggregation within the endothelium prevent propagation of platelet aggregation beyond the area of vascular injury; these mechanisms include endothelial NO, prostacyclin, and ectonucleotidase [7, 10].
Thrombin Generation in the Cell-Based Model of Coagulation
The second stage of hemostasis occurs following activation of coagulation through factor VII activation by tissue factor (TF), which is released from damaged vascular endothelium and is expressed by monocytes, macrophages, neutrophils, activated endothelial cells, platelet microparticles, and smooth muscle [6, 7]. Factor X is activated by VIIa-Va-TF complex and combines with FVa activating FII (prothrombin) , resulting in a small amount of thrombin (IIa) production [13]. Thrombin causes activation of platelets, which is demonstrated by expression of negatively charged phospholipids on their surfaces, release and activation of FV from the dense granules, and activation of FXIII. In addition, thrombin releases FVIIIa from vWF, which is also expressed on the platelet surface. FIXa and FVIIIa complex on the negatively charged phospholipids of the activated platelet surface and activate FXa. The activated platelets expressing the complex FXa-Va then act on FII producing thrombin, resulting in fibrin monomer production, which is polymerized by factor XIII [5, 6, 13].
Modulation of coagulation is carried out by antithrombin (AT), which inhibits FXa, FIIa, and TFPI, which then inhibits TF-FVIIa complex, FXa, and protein C, which itself is activated by thrombin when it binds to the membrane receptor, thrombomodulin, and endothelial cell protein C receptor. This complex subsequently binds to protein S and inhibits FVa and FVIIIa [8].
Fibrinolysis (Clot Dissolution) and Modulation
The third stage of hemostasis, fibrinolysis, involves dissolution of the fibrin clot. Production of thrombin activates tissue plasminogen activator (tPA), the most important activator of plasminogen, which is subsequently converted to plasmin. Plasmin degrades the polymerized fibrin clot into small fragments known as d-dimers [14]. Modulation of fibrinolysis is carried out by decreasing plasminogen availability, either through degradation by thrombin-activatable fibrinolysis inhibitor (TAFI) or inhibition by plasminogen activator inhibitor (PAI) [14]. In addition, α2-macroglobulin inhibits plasmin activity [14].
Hemostasis Measurement
Hemostasis can be measured by determining the concentration or amount of participants (coagulation and fibrinolytic factors, platelets), interaction of the participants in a plasma-based system (activated partial thromboplastin time [aPTT], prothrombin time [PT] converted to the international normalized ratio [INR], thrombin clotting time [TCT], and platelet aggregometry) or in whole blood (d-dimer, activated clotting time [ACT], viscoelastic testing of clot formation, i.e., thromboelastography or thromboelastometry). The plasma-based assays have been criticized for not reflecting in vivo hemostasis due to the absence of cellular components, which are major participants in the process. An overview of hemostatic testing can be found elsewhere [5, 14, 15].
Developmental Hemostasis: Differences Between Children and Adults
Children differ from adults in the participants and the inhibitory mechanisms of hemostasis, thereby influencing the epidemiology, clinical manifestations, and management of thrombosis.
Platelets
Platelets in neonates and children are present in the same number and size, with the same receptors [16–18]. (The cited papers do not compare neonates or children to adults.) However, depending on the method of testing, neonatal platelet function may be decreased or increased. Neonatal platelets have decreased function when measured by granule secretion [19, 20]; aggregometry using the agonists epinephrine, collagen, ADP, and thromboxane [21]; and activation markers P selectin and CD63 [19, 20, 22]. These differences in function are due to decreased numbers of fibrinogen binding sites exposed on GPIIb-IIIa, impaired receptor-mediated signal transduction at thromboxane receptor [23], impairment of calcium mobilization [24], and decreased adrenergic receptors (epinephrine) [25]. Neonatal platelets have increased function when demonstrated by whole blood assays measuring platelet function by PFA 100 [26] and adhesiveness/aggregation on extracellular matrix-coated cone and plate analyzer [18]. These results suggest enhanced platelet and vessel wall interaction, perhaps maximized by other neonatal differences in whole blood composition, for instance, increased hematocrit, red cell volume, vWF concentration, and large-molecular-weight forms [26–28].
Coagulation Proteins
The integral proteins in the hemostatic system (factors XII, XI, X, IX, VIII, VII, V, II, and fibrinogen) are synthesized by the fetus [29, 30]. Levels of the proteins approach adult levels at different times during childhood; the contact factors, FXII and FXI, and vitamin K-dependent factors (FII, FVII, FIX, FX) reach adult levels by about age 5 [31]. In newborns, fibrinogen levels are quantitatively the same as adults, but the protein is qualitatively different, with increased glycosylation (sialic acid) and phosphorus content and decreased rate of polymerization with the ratio of function to quantity being increased compared to children and adults [32]. Inhibitory proteins, proteins C, S, and AT, are decreased and approach adult levels at 3–6 months of age [33]. α2-Macroglobulin levels are increased until adolescence when they approach adult levels [33]. The amount of thrombin produced is decreased in neonates and children [30, 34]. However, despite the reduced thrombin generation demonstrated in infants and neonates, the levels of coagulation inhibitors are normally less than in adults, and thus the lag time to thrombin generation is much shorter [35, 36]. Tissue factor pathway inhibitor (free form) is decreased throughout childhood compared to adults [30].
Fibrinolysis
Regarding fibrinolysis, children ages 1–18 years and adults have similar levels of tPA, PAI, and TAFI [37]. Compared to adults, plasminogen levels are decreased until 6 months of age, with slow activation kinetics by tPA [31]. Using venous occlusion technique, adolescents demonstrate decreased levels of tPA antigen and increased activity of PAI-1 resulting in ex vivo increased clot lysis times [38], suggesting decreased fibrinolysis in children. Other laboratory techniques suggest increased fibrinolysis, including increased d-dimer levels in neonates and children, and by functional fibrinolysis testing in neonates using thromboelastometry [30, 39, 40].
Vascular Endothelium
Studies in rabbits document structural and functional differences between prepubertal and adult animals in the aortic and inferior vena cava endothelium [41, 42], specifically in endothelial function (increased inhibition of thrombin activity), extracellular matrix structure, proteoglycan distribution (increased chondroitin and heparin sulfate), glycosaminoglycan content (increased chondroitin and dermatan sulfate), and function [41, 42]. The endothelium of newborn mice phenotypically resembles dysfunctional endothelial cells of vascular disease, with prominent stress fibers and marked inhibition of endothelial dilation [43].
Differences in Hemostatic Testing in Children
Differences in components of hemostasis in children result in differences in hemostatic testing. The aPTT and PT/INR are prolonged in newborns up to late adolescence and remain slightly higher but significantly elevated compared to adults [30]. The TCT is increased but approaches adult levels by 6 years of age [30]. Global hemostasis testing using either thromboelastography or thromboelastometry in neonates and children demonstrates faster initiation of coagulation despite the increased aPTT/PT, but with varying results between studies for clot firmness [28, 44–48]. The plasma-based assays have been criticized for not reflecting in vivo hemostasis due to the absence of cellular components. An overview of hemostatic testing can be found elsewhere [5, 14, 15].
The differences in hemostasis add additional confounding variables to the epidemiology and management of children with or at risk for thrombosis. Hemostatic testing results are often further altered when aPL increases aPTT and/or PT/INR. Children with APS are at increased risk for thrombosis [49–51]; whether the risk is greater than adults is unknown [52–54].
Thrombosis Risk Assessment (Genetic and Extrinsic)
Assessment of Virchow’s triad of stasis, vascular injury, and hypercoagulability is inherent in the assignment of thrombotic risk. The antithrombotic milieu in a child slowly acquires characteristics of an adult with the onset of puberty. Compared to adults, children are relatively protected from thrombosis because of the decreased potential for thrombin generation, increased α2-macroglobulin, and the antithrombotic potential of the vascular wall. Children may acquire additional risk factors in adolescence, including smoking, birth control pills, and pregnancy. Preexisting risk factors such as obesity and dyslipoproteinemia, which may have been present earlier, have an amplified effect. As the vascular endothelium decreases in antithrombotic potential, vascular injury from play activity and sports may impose additional risk.
Antiphospholipid antibodies coincident with inherited thrombophilia (for isolated or combinations of genetic mutations) are associated with increased relative risk of thrombosis. The increased risk of thrombosis associated with aPL and factor V Leiden mutations in adults and children has been discussed elsewhere [55–58].
Clinical and Laboratory Features of Antiphospholipid Syndrome in Children
Antiphospholipid syndrome plays an important role in pediatric venous and arterial thromboembolic events, including stroke [59, 60], but is rare among all patients diagnosed as having APS: onset prior to the age of 15 occurred in only 2.8% of all patients with APS [61]. Pediatric and neonatal APS have been addressed by few studies [62–64].
Although the criteria of obstetric morbidity are not applicable to prepubertal children, and despite lack of validation in children and neonates, the same diagnostic criteria are used for classification of pediatric APS [65]. Nonetheless, pediatric APS presents with symptoms similar to adults [66]. Katzav et al. [67] showed that patients with childhood-onset APS presented with more episodes of chorea and jugular vein thrombosis than did adults [67]. Arterial thrombosis is more common in children [63, 68]. In a cohort of 28 children with APS [69], the most common initial manifestations were venous thrombosis, stroke, and thrombocytopenia. Lupus anticoagulant was detected in 96% of those tested. Seven of the 24 patients with vascular thrombotic events had recurrences. Hereditary thrombophilia was more common in children who experienced a single episode of APS (8 [53.3%] of 15 patients) than in those who experienced recurrences (2 [28.6%] of 7 patients). However, only two patients in the latter group (28.6%) received anticoagulants after the first manifestation, compared with 12 (70.6%) of the 17 patients without recurrences. Infants with perinatal stroke usually display a monophasic disease, and other manifestations of APS do not develop later.
Based on very small studies, levels of IgA anticardiolipin (aCL) antibody are lower in children with APS than in adults, whereas IgG anti-β2-glycoprotein-I (aβ2GPI) levels are highest in preschool children [69, 70].
An international registry of pediatric APS , established in 2004 [63], currently documents standardized clinical, laboratory, and therapeutic data of 140 children with definite APS seen at 24 pediatric university centers. Thrombotic events include venous thrombosis in 60%, arterial thrombosis in 32%, small vessel thrombosis in 6%, and mixed arterial and venous thrombosis in 2% of the first 121 patients [63]. During a follow-up period of 6.1 years, 19% developed recurrent thrombosis [63] and 5% developed catastrophic APS.
Perinatal stroke is a special entity in children with APS [71, 72]. Perinatal arterial stroke (PAS) may result from focal arterial or venous thrombosis or emboli occurring between 20 weeks of fetal life and the 28th postnatal day. Diagnosis may be delayed; reported prevalence is 1:4000–5000 live births [72]. Risk factors may relate to both fetal and neonatal disorders as well as maternal and placental conditions. The relative roles of genetic and acquired thrombophilia in the pathogenesis of PAS are controversial. Positive aPL tests can be found in both neonates with PAS or their mothers, or both [60]. Berkun et al. [72] presented a cohort of 12 neonates with cerebral thromboembolism and persistent aPL whom they followed prospectively with repeated antibody testing. In 10/12 cases aPL levels decreased to normal within a median of 2.5 years. Notably, following the diagnosis of perinatal cerebral event, 11 of the patients received no anticoagulant therapy; a single infant who suffered from perinatal cerebral sinus venous thrombosis was treated with low-molecular-weight heparin (LMWH) until the age of 6 months. In this cohort, maternal aPL was detected in only two cases, not necessarily correlating with the antibodies found among the affected infants. Similarly, within an Israeli PAS cohort of eight mother-infant pairs [60], aPL discordance was noted between mothers and their offspring: in three cases, only the mother had aPL; in four, only the infant; and in one, both mother and infant. These findings indicate that maternal antibodies are not the only pathogenic factor for neonatal APS. An important finding was the absence of recurrent thrombosis or other APS manifestations, despite lack of prolonged anticoagulation; also, there was gradual disappearance of aPL. Timing of perinatal brain injury introduces multiple, often competing, factors in brain maturation and development that will ultimately determine outcome.
Epidemiologic pediatric studies show that, from 1 year to puberty, pediatric patients are less prone to thrombosis than are adults. Low titer and transient aPL [72] are frequent within the pediatric population, but thrombosis is rare. Overall, perinatal stroke in children with aPL may not require anticoagulant therapy unless other risk factors prevail.
A few registry-based studies suggest that neurological manifestations occur in 16–22% of pediatric APS cases [63, 73]. They include thrombosis (arterial and venous); immune-mediated inflammatory change; and secondary complications or symptoms, for instance, seizures, headaches, focal neurological deficits/signs, and cognitive changes. Psychiatric symptoms, including psychosis and mood disturbance, have also been described [63, 74].
Regarding lupus-associated APS, the most common neurological manifestation is thrombosis, including both arterial stroke and venous sinus thrombosis [74]. The 121-patient pediatric APS registry found a 16% prevalence of headache, 9% of cognitive dysfunction, and 10% of psychosis [75]. Comparison of lupus patients with and without aPL suggests an association between aβ2GPI and neuropsychiatric manifestations (p = 0.02). Among 32 patients with both primary and other autoimmune disease-associated aPL, and that defined neurological manifestations only as epilepsy, chorea, or aseptic meningitis, seizures were the most common neurological manifestation, occurring in five (16%) [73].
In studies documenting the rate of aPL positivity in neurologic cohorts, there is no increase of aPL in pediatric headache patients compared to controls [76]. Approximately 30% of 142 consecutive pediatric epilepsy patients in one cross-sectional cohort study were positive for aPL, but selection bias and lack of controls in this study limit generalizability of the findings [77]. In another study of 80 pediatric epilepsy patients, only three (3.8%) were positive for aPL, with aCL found in one (1.3%) [78].
In pediatric APS, neurological complications are common and may be significant. Future studies should provide detailed documentation of functional and structural neurological changes , including detailed cognitive and neurological assessments and MRI studies using advanced metrics.
Prevention and Treatment of Antiphospholipid Syndrome in Children
Assessment of the risk posed by acquired or extrinsic factors is essential to treatment decisions [79, 80]. The treatment of “at-risk” children may not always include anticoagulants but may include dietary changes, behavior modification, and/or treatment of associated conditions (none of which, however, are evidence-based). Endothelial activation and endothelial damage associated with inflammation may shift the balance toward thrombosis. Thus, treatment of an underlying disease may be of benefit. In an otherwise-healthy, low-risk child with a postinfectious event, this prothrombotic situation may be transient; however, in children with underlying rheumatic diseases, anticoagulation therapy may need to be continued until disease activity is quiescent. Deitcher and Carman [81], studying adults, suggested that “lifelong” and “long-term” treatment are different, the latter implying that the issue will be revisited. Risk stratification and individualized therapy determine how long a patient is treated.
In children, identification of risk factors and provision of preventive health, and short-term treatment of transient events, may be more important than in adults because of developmental hemostasis [82].
A recent European project, SHARE (Single Hub and Access Point for Pediatric Rheumatology in Europe), systematically evaluated published data on APS in pediatric populations to provide 14 evidence-based recommendations for the diagnosis and treatment. When available, these recommendations will help practicing physicians and facilitate improvement and uniformity of care of children with APS [83].
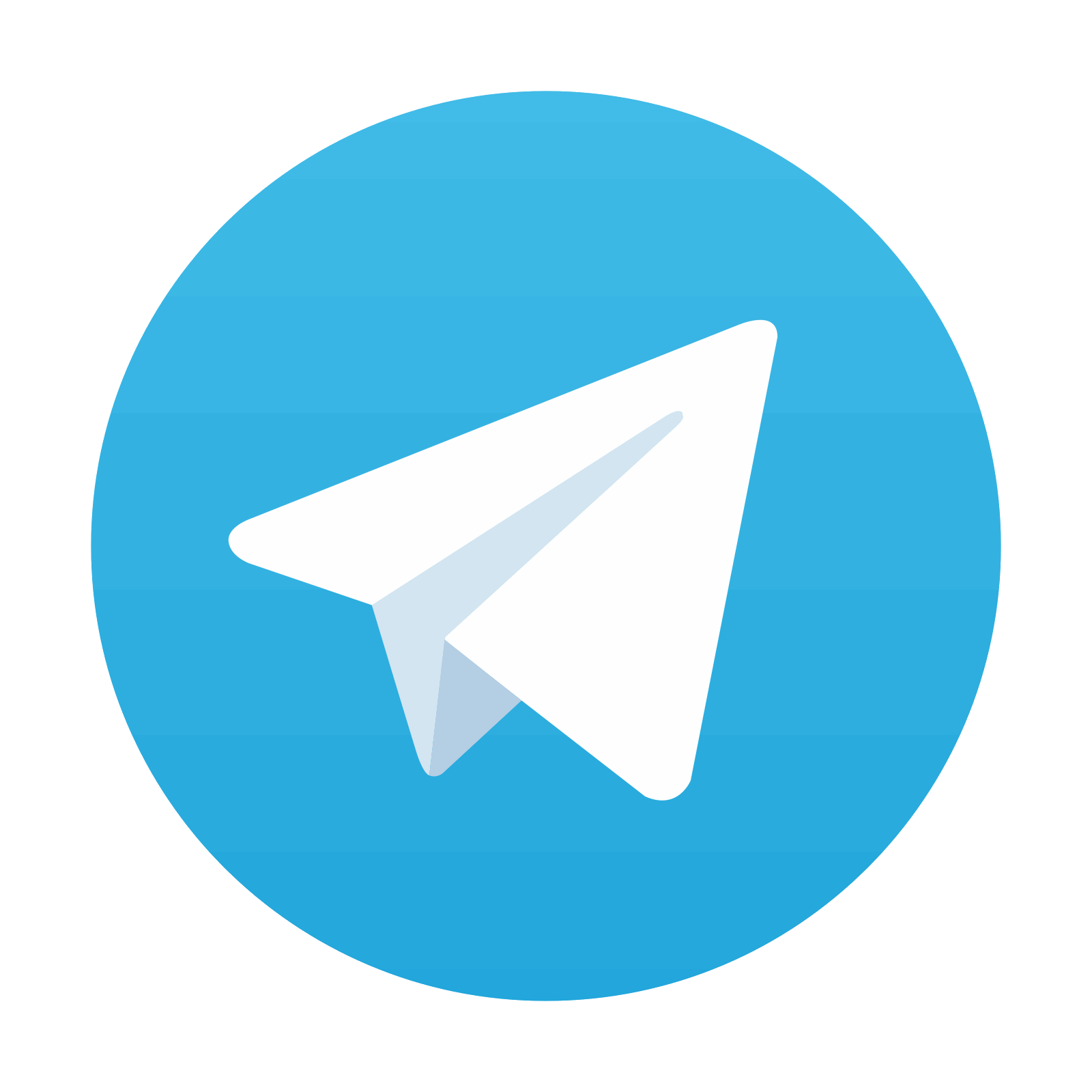
Stay updated, free articles. Join our Telegram channel

Full access? Get Clinical Tree
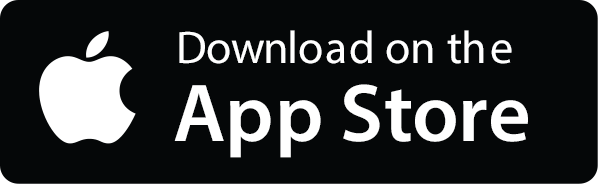
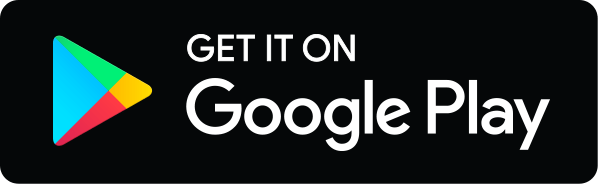