- 1.1 Introduction
- 1.2 Key molecules
- 1.2.1 Molecules recognized by immune systems
- 1.2.2 Recognition molecules
- 1.2.3 Accessory molecules
- 1.2.4 Effector molecules for immunity
- 1.2.5 Receptors for effector functions
- 1.2.6 Adhesion molecules
- 1.2.1 Molecules recognized by immune systems
- 1.3 Functional basis of innate responses
- 1.3.1 Endothelial cells
- 1.3.2 Neutrophil polymorphonuclear leucocytes
- 1.3.3 Macrophages
- 1.3.4 Dendritic cells
- 1.3.5 Complement
- 1.3.6 Antibody-dependent cell-mediated cytotoxicity
- 1.3.7 Natural killer cells
- 1.3.1 Endothelial cells
- 1.4 Functional basis of the adaptive immune responses
- 1.4.1 Antigen processing
- 1.4.2 T cell mediated activation responses
- 1.4.3 Antibody production
- 1.4.1 Antigen processing
- 1.5 Physiological outcomes of immune responses
- 1.5.1 Killing of target cells (virally infected/tumour cells)
- 1.5.2 Direct functions of antibody
- 1.5.3 Indirect functions of antibody
- 1.5.4 Regulation
- 1.5.1 Killing of target cells (virally infected/tumour cells)
- 1.6 Tissue damage caused by the immune system
- 1.6.1 Inflammation: a brief overview
- 1.7 Organization of the immune system: an overview
- 1.8 Conclusions
Visit the companion website at www.immunologyclinic.com to download cases on these topics.
1.1 Introduction
The immune system evolved as a defence against infectious diseases. Individuals with markedly deficient immune responses, if untreated, succumb to infections in early life. There is, therefore, a selective evolutionary pressure for a really efficient immune system. Although innate systems are fast in response to pathogens, the evolution to adaptive responses provided greater efficiency. However a parallel evolution in pathogens means that all species, plants, insects, fish, birds and mammals, have continued to improve their defence mechanisms over millions of years, giving rise to some redundancies as well as resulting in apparent complexity. The aim of this chapter is to provide an initial description of the molecules involved, moving onto the role of each in the immune processes rather than the more traditional sequence of anatomical structure, cellular composition and then molecular components. It is hoped that this gives a sense of their relationship in terms of immediacy and dependency as well as the parallel evolution of the two immune systems. An immune response consists of five parts:
Innate immunity, though phylogenetically older and important in terms of speed of a response, is less efficient. Humoral components (soluble molecules in the plasma) and cells in blood and tissues are involved. Such responses are normally accompanied by inflammation and occur within a few hours of stimulation (Table 1.1).
Table 1.1 Components of innate and adaptive immunity
Features | Innate | Adaptive |
---|---|---|
Foreign molecules recognized | Structures shared by microbes, recognized as patterns (e.g. repeated glycoproteins) PAMPs | Wide range of very particular molecules or fragments of molecules on all types of extrinsic and modified self structures |
Nature of recognition receptors | Germline encoded – limited PRRs | Somatic mutation results in wide range of specificities and affinities |
Speed of response | Immediate | Time for cell movement and interaction between cell types |
Memory | None | Efficient |
Humoral components | Complement components | Antibodies |
Cellular components | Dendritic cells, neutrophils, macrophages, NK cells, NKT cells, B1 cells, epithelial cells, mast cells | Lymphocytes – T (Th1, Th2, Th17, T regs) B |
iNKT cells, γδ T cells |
Adaptive immune responses are also divided into humoral and cellular responses. Adaptive humoral responses result in the generation of antibodies reactive with a particular antigen. Antibodies are proteins with similar structures, known collectively as immunoglobulins (Ig). They can be transferred passively to another individual by injection of serum. In contrast, only cells can transfer cellular immunity. Good examples of cellular immune responses are the rejection of a graft by lymphoid cells as well as graft-versus-host disease, where viable transferred cells attack an immunologically compromised recipient that is unable to fight back.
Antibody-producing lymphocytes, which are dependent on the bone marrow, are known as B cells. In response to antigen stimulation, B cells will mature to antibody-secreting plasma cells. Cellular immune responses are dependent on an intact thymus, so the lymphocytes responsible are known as thymus-dependent (T) cells. The developmental pathways of both cell types are fairly well established (Fig. 1.1).
Fig. 1.1 Development of different types of blood from a pluripotential stem cell in the bone marrow. The developmental pathway for natural killer (NK) cells is shown separately because it is thought NK cells may develop in both the thymus and the bone marrow.
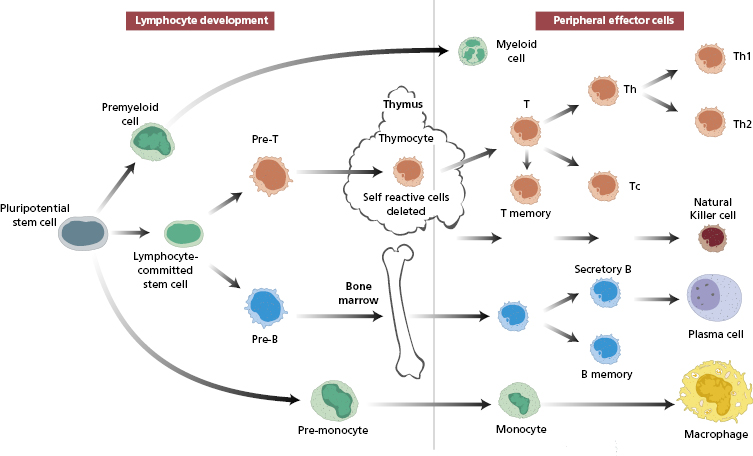
The recognition phase is common to both adaptive and innate immunity. It involves professional cells, known as classical dendritic cells, that recognize general pathogen features or specific antigenic molecules, process the antigens and present antigen fragments to the other cells of the immune systems as well as initiating non-specific inflammation to the pathogen. In the effector phase, neutrophils and macrophages (innate immunity) and antibodies and effector T lymphocytes (adaptive immunity) eliminate the antigen.
In terms of disease, like other organs, the immune system may fail (immunodeficiency), may be come malignant (lymphoid malignancies) or produce aberrant responses (such as in autoimmunity or allergy). This chapter describes the normal immune system in order to lay the basis for discussing these ways in which it can go wrong and so cause disease.
1.2 Key molecules
Many types of molecules play vital roles in both phases of immune responses; some are shared by both the innate and the adaptive systems. Antigens are substances that are recognized by immune components. Detection molecules on innate cells recognize general patterns of ‘foreignness’ on non-mammalian cells, whereas those on adaptive cells are specific for a wide range of very particular molecules or fragments of molecules. Antibodies are not only the surface receptors of B cells (BCRs) that recognize specific antigens, but, once the appropriate B cells are activated and differentiate into plasma cells, antibodies are also secreted into blood and body fluids in large quantities to prevent that antigen from causing damage. T cells have structurally similar receptors for recognizing antigens, known as T-cell receptors (TCRs). Major histocompatibility complex (MHC) molecules provide a means of self-recognition and also play a fundamental role in T lymphocyte effector functions. Effector mechanisms are often dependent on messages from initiating or regulating cells; soluble mediators, which carry messages between cells, are known as interleukins, cytokines and chemokines.
1.2.1 Molecules recognized by immune systems
Foreign substances are recognized by both the innate and adaptive systems, but in different ways, using different receptors (see section 1.2.2). The innate system is activated by ‘danger signals’, due to pattern recognition receptors (PRRs) on dendritic cells recognizing conserved microbial structures directly, often repeated polysaccharide molecules, known as pathogen-associated molecular patterns (PAMPs). Toll-like receptors (receptors which serve a similar function to toll receptors in drosophila) make up a large family of non-antigen-specific receptors for a variety of individual bacterial, viral and fungal components such as DNA, lipoproteins and lipopolysaccharides. Activation of dendritic cells by binding to either of these detection receptors leads to inflammation and subsequently activation of the adaptive system.
Phagocytic cells also recognize particular patterns associated with potentially damaging materials, such as lipoproteins and other charged molecules or peptides.
Traditionally, antigens have been defined as molecules that interact with components of the adaptive system, i.e. T- and B-cell recognition receptors and antibody. An antigenic molecule may have several antigenic determinants (epitopes); each epitope can bind with an individual antibody, and a single antigenic molecule can therefore provoke many antibody molecules with different binding sites. Some low-molecular-weight molecules, called haptens, are unable to provoke an immune response themselves, although they can react with existing antibodies. Such substances need to be coupled to a carrier molecule in order to have sufficient epitopes to be antigenic. For some chemicals, such as drugs, the carrier may be a host (auto) protein. The tertiary structure, as well as the amino acid sequence, is important in determining antigenicity. Pure lipids and nucleic acids are poor antigens, although they do activate the innate system and can be inflammatory.
Antigens are conventionally divided into thymus-dependent and thymus-independent antigens. Thymus-dependent antigens require T-cell participation to provoke the production of antibodies; most proteins are examples. Thymus-independent antigens require no T-cell cooperation for antibody production; they directly stimulate specific B lymphocytes by virtue of their ability to cross-link antigen receptors on the B-cell surface, produce predominantly IgM and IgG2 antibodies and provoke poor immunological memory. Such antigens include bacterial polysaccharides, found in bacterial cell walls. Endotoxin, another thymus-independent antigen, not only causes specific B-cell activation and antibody production but also acts as a stimulant for all B cells regardless of specificity.
Factors other than the intrinsic properties of the antigen can also influence the quality of the immune response (Table 1.2). Substances that improve an immune response to a separate, often rather weak, antigen are known as adjuvants. The use of adjuvants in humans, important in vaccines against infective agents and tumours, is discussed in section 7.3.2.
Table 1.2 Factors influencing the immune response to an antigen, i.e. its immunogenicity
1 Nature of molecule: Protein content Size Solubility |
2 Dose: Low doses provoke small amounts of antibody with high affinity and restricted specificity Moderate doses provoke large amounts of antibody but mixed affinity and broad specificity High doses provoke tolerance |
3 Route of entry: ID, IM, SC→regional lymph nodes IV→spleen Oral→Peyer’s patches Inhalation→bronchial lymphoid tissue |
4 Addition of substances with synergistic effects, e.g. adjuvants, |
5 Genetic factors of recipient animal: Species differences Individual differences |
ID, Intradermal injection; IM, intramuscular injection; IV, intravenous injection; SC, subcutaneous injection.
Superantigen is the name given to those foreign proteins which are not specifically recognized by the adaptive system but do activate large numbers of T cells regardless of specificity, via direct action with an invariant part of the TCR (see section 2.4.2).
Self-antigens are not recognized by dendritic cells, so inflammation and co-stimulation of T cells (see section 1.4.1) is not induced. There are mechanisms to control any aberrant adaptive responses to self-antigens, by prevention of production of specific receptors and regulation of the response if the immune system is fooled into responding (see Chapter 5, Autoimmunity).
1.2.2 Recognition molecules
There are several sets of detection molecules on dendritic cells (Table 1.3): pattern recognition receptors (PRRs), such as Toll-like receptors, as well as chemotactic receptors and phagocytic receptors. PRRs may be soluble or attached to cell membranes. Mannan binding lectin is a protein that binds sugars on microbial surfaces; if attached to a macrophage, it acts as a trigger for phagocytosis and, if soluble, it activates the complement cascade resulting in opsonization. Others belonging to this family are less well defined.
Table 1.3 Markers on dendritic cells
Immature dendritic cells | Mature myeloid dendritic cells | |
---|---|---|
Function | Antigen capture | Antigen presentation to immature T cells for specific differentiation |
Co-stimulatory molecule expression, e.g. CD80, CD86 | Absent or low | ++ |
Adhesion molecules, e.g. ICAM-1 | Absent or low | ++ |
Cytokine receptors, e.g. IL-12R | Absent or low | ++ |
Pattern recognition receptors (PRRs), e.g. mannose receptor | ++ | − |
MHC class II: | ||
turnover | Very rapid | Persist >100 h |
density | Reduced (approx. 1 × 106) | Very high (approx. 7 × 106) |
ICAM-1, Intercellular adhesion molecule-1.
Toll-like receptors (TLRs) are part of this family too. These are evolutionarily conserved proteins found on macrophages, dendritic cells and neutrophils. At least ten different TLRs are found in humans, each TLR recognizing a range of particular motifs on pathogens, such as double-stranded RNA of viruses (TLR3), lipopolysaccharides of Gram-negative bacterial cell walls (TLR4), flagellin (TLR5) and bacterial DNA (TLR9), all highly conserved motifs unique to microorganisms. Upon binding to their ligands, TLRs induce signal transduction, via a complex cascade of intracellular adaptor molecules and kinases, culminating in the induction of nuclear factor kappa B transcription factor (NFκB)-dependent gene expression and the induction of pro-inflammatory cytokines (Fig. 1.2). The clinical consequences of a defective TLR pathway are discussed in section 3.4.1 (see Box 1.1 in this chapter also).
Fig. 1.2 Sequential cellular events induced by engagement of Toll-like receptors on dendritic cells neutrophils and macrophages by microbial ligands (TRAF, TNF receptor-associated factor; IKB, inhibitor kappa B; MAPK, mitogen-activated protein kinase; IRAK, interleukin-1 receptor-associated kinase).
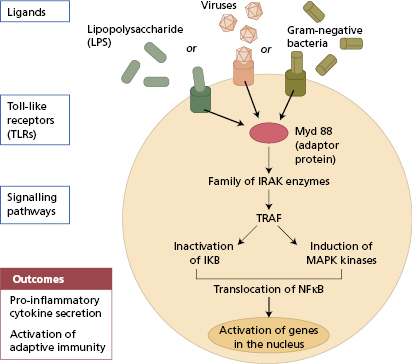
In humans, deficiency of IRAK-4 (interleukin-1 receptor-associated kinase) or MyDD88, key intracellular molecules responsible for TLR signal transduction (Fig. 1.2) is associated with recurrent pyogenic bacterial infections accompanied by failure to mount an appropriate acute-phase response (Case 3.6).
Mice lacking TLR4 are exceptionally susceptible to infection with Gram-negative bacteria
CD1 molecules are invariant proteins (MHC-like and associated with β2-microglobulin – see later), which are present on dendritic and epithelial cells. CD1 combine with lipids, which are poor antigens and not usually well presented to the adaptive immune system, and so act as recognition molecules for the intestine and other microbial rich surfaces. CD1 present lipids to the immune cells of the gut in particular, namely non-MHC-restricted natural killer (NKT) cells and γδ T cells in the epithelium.
Antigenic epitopes, having been processed by dendritic cells, are recognized by cells of the adaptive system by means of specific receptors. Each T cell, like B cells, is pre-committed to a given epitope. It recognizes this by one of two types of TCRs, depending on the cell’s lineage and thus its effector function. T cells have either αβTCR [a heterodimer of alpha (α) and beta β) chains] or γδTCR [a heterodimer of gamma γ and delta (δ) chains]. αβTCR cells predominate in adults, although 10% of T cells in epithelial structures are of the γδTCR type. In either case, TCRs are associated with several transmembrane proteins that make up the cluster differentiation 3 (CD3) molecule (Fig. 1.3), to make the CD3–TCR complex responsible for taking the antigen recognition signal inside the cell (signal transduction). Signal transduction requires a group of intracellular tyrosine kinases (designated p56 lck, p59 fyn, ZAP 70) to join with the cytosolic tails of the CD3–TCR complex and become phosphorylated. Nearby accessory molecules, CD2, LFA-1, CD4 and CD8, are responsible for increased adhesion (see section 1.2.6) but are not actually involved in recognizing presented antigenic fragments.
Fig. 1.3 Diagram of the structure of the T-cell receptor (TCR). The variable regions of the alpha (α) and beta (β) chains make up the T idiotype, i.e. antigen/peptide binding region. The TCR is closely associated on the cell surface with the CD3 protein that is essential for activation.
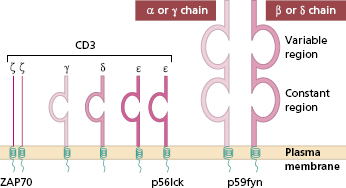
The genes for TCR chains are on different chromosomes: β and γ on chromosome 7 and α and δ on chromosome 14. Each of the four chains is made up of a variable and a constant domain. The variable regions are numerous (although less so than immunoglobulin variable genes; they are joined by D and J region genes to the invariant (constant) gene by recombinases, RAG1 and RAG2, the same enzymes used for making antigen receptors on B cells (BCRs) and antibodies (section 1.4.1). The diversity of T-cell antigen receptors is achieved in a similar way for immunoglobulin, although TCRs are less diverse since somatic mutation is not involved; perhaps the risk of ‘self recognition’ would be too great. The diversity of antigen binding is dependent on the large number of V genes and the way in which these may be combined with different D and J genes to provide different V domain genes. The similarities between TCRs and BCRs led to the suggestion that the genes evolved from the same parent gene and both are members of a ‘supergene’ family. Unlike immunoglobulin, TCRs are not secreted and are not independent effector molecules.
A particular TCR complex recognizes a processed antigenic peptide in the context of MHC class I or II antigens (section 1.4.1) depending on the type of T cell; helper T cells recognize class II with antigen, and this process is enhance by the surface accessory protein CD4 (see later) and intracellular signals. Cytotoxic T cells (CTL/Tc) recognize antigens with class I (see section 1.3.1) and use CD8 accessory molecules for increased binding and signalling. Since the number of variable genes available to TCRs is more limited, reactions with antigen might not be sufficient if it were not for the increased binding resulting from these accessory mechanisms. Recognition of processed antigen alone is not enough to activate T cells. Additional signals, through soluble cytokines (interleukins), are needed; some of these are generated during ‘antigen processing’ (see Antigen processing, section 1.4.1).
Major histocompatibility complex molecules (MHC) were originally known as ‘histocompatibility antigens’ because of the vigorous reactions they provoked during mismatched organ transplantation. However, these molecules are known to play a fundamental role in immunity by presenting antigenic peptides to T cells. Histocompatibility antigens in humans [known as human leucocyte antigens (HLA)] are synonymous with the MHC molecules. MHC molecules are cell-surface glycoproteins of two basic types: class I and class II (Fig. 1.5). They exhibit extensive genetic polymorphism with multiple alleles at each locus. As a result, genetic variability between individuals is very great and most unrelated individuals possess different MHC (HLA) molecules. This means that it is very difficult to obtain perfect HLA matches between unrelated persons for transplantation (see Chapter 8). The extensive polymorphism in MHC molecules is best explained by the need of the immune system to cope with an ever-increasing range of pathogens adept at evading immune responses (see Chapter 2).
The TCR of an individual T cell will only recognize antigen as a complex of antigenic peptide and self-MHC (Fig. 1.4). This process of dual recognition of peptide and MHC molecule is known as MHC restriction, since the MHC molecule restricts the ability of the T cell to recognize antigen (Fig. 1.4). The importance of MHC restriction in the immune response was recognized by the award of the Nobel Prize in Medicine to Peter Doherty and Rolf Zinkernagel, who found that virus-specific CTLs would only kill cells of the same particular allelic form of MHC molecule.
Fig. 1.4 MHC restriction of antigen recognition by T cells. T cells specific for a particular peptide and a particular MHC allele will not respond if the same peptide were to be presented by a different MHC molecule as in (ii) or as in (iii) if the T cell were to encounter a different peptide. APC, Antigen-presenting cell; TCR, T-cell receptor.
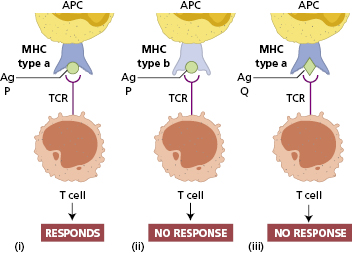
MHC class I antigens are subdivided into three groups: A, B and C. Each group is controlled by a different gene locus within the MHC region on chromosome 6 (Fig. 1.6) in humans (different in mice). The products of the genes at all three loci are chemically similar. All MHC class I antigens (see Fig. 1.5) are made up of an α heavy chain, controlled by a gene in the relevant MHC locus, associated with a smaller chain called β2-microglobulin, controlled by a gene on chromosome 12. The differences between individual MHC class I antigens are due to variations in the α chains; the β2-microglobulin component is constant. The detailed structure of class I antigens was determined by X-ray crystallography. This shows that small antigenic peptides (approx. nine amino acids long) can be tightly bound to a groove produced by the pairing of the two extracellular domains (α1 and α2) of the α chain. The affinity (tightness of binding) of individual peptide binding depends on the nature and shape of the groove, and accounts for the MHC restriction mentioned earlier.
Fig. 1.5 Diagrammatic representation of MHC class I and class II antigens. β2m, β2-microglobulin; CHO, carbohydrate side chain.
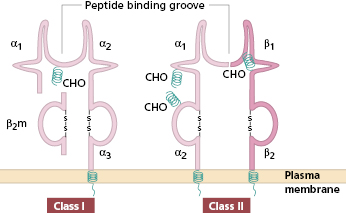
Fig. 1.6 Major histocompatibility complex on chromosome 6; class III antigens are complement components. TNF, Tumour necrosis factor.
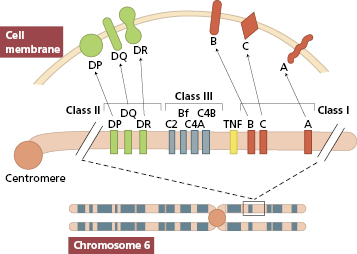
MHC class II antigens have two heavy chains, α and β, both coded for by genes in the MHC region of chromosome 6. The detailed structure of MHC class II antigens was also determined by X-ray crystallography. It has a folded structure similar to class I antigens with the peptide-binding groove found between the α and β chains (see Fig. 1.5). Whereas most nucleated cells express class I molecules, expression of class II molecules is restricted to a few cell types: dendritic cells, B lymphocytes, activated T cells, macrophages, inflamed vascular endothelium and some epithelial cells. However, other cells (e.g. thyroid, pancreas, gut epithelium) can be induced to express class II molecules under the influence of interferon (IFN)-γ released during inflammation. In humans, there are three groups of variable class II antigens: the loci are known as HLA-DP, HLA-DQ and HLA-DR.
In practical terms, there are different mechanisms by which antigens in different intracellular compartments can be captured and presented to CD4+ or CD8+ T cells (Fig. 1.7). Endogenous antigens (including viral antigens that have infected host cells) are processed by the endoplasmic reticulum and presented by MHC class I-bearing cells exclusively to CD8+ T cells. Prior to presentation on the cell surface, endogenous antigens are broken down into short peptides, which are then actively transported from the cytoplasm to endoplasmic reticulum by proteins. These proteins act as a shuttle and are so named ‘transporters associated with antigen processing’ (TAP-1 and TAP-2). TAP proteins (also coded in the MHC class II region) deliver peptides to MHC class I molecules in the endoplasmic reticulum, from whence the complex of MHC and peptide is delivered to the cell surface. Mutations that affect function in either TAP gene prevent surface expression of MHC class I molecules.
In contrast, exogenous antigens are processed by the lysosomal route and presented by MHC class II antigens to CD4+ T cells (Fig. 1.7). As with MHC class I molecules, newly synthesized MHC class II molecules are held in the endoplasmic reticulum until they are ready to be transported to the cell surface. Whilst in the endoplasmic reticulum, class II molecules are prevented from binding to peptides in the lumen by a protein known as MHC class II-associated invariant chain. The invariant chain also directs delivery of class II molecules to the endosomal compartment where exogenous antigens are processed and made available for binding to class II molecules.
The MHC class III region (see Fig. 1.6) contains genes encoding proteins that are involved in the complement system (see section 1.4.1): the early components C4 and C2 of the classical pathway and factor B of the alternative pathway. Some inflammatory proteins, e.g. tumour necrosis factor (TNF), are also encoded in adjacent areas. Invariant MHC-like proteins, such as CD1 lipid-recognition receptors (see earlier), are not coded for on chromosome 6, despite being associated with β2-microglobulin.
In contrast to TCRs, the antigen receptors on B cells (BCRs) are surface-bound immunoglobulin molecules that can be secreted as soluble molecules. As with TCRs, they have predetermined specificity for epitopes and are therefore extremely diverse. The immune system has to be capable of recognizing all pathogens, past and future. Such diversity is provided by the way in which all three types of molecules, TCR, BCR and antibody, are produced.
The basic structure of the immunoglobulin molecule is shown in Fig. 1.8. It has a four-chain structure: two identical heavy (H) chains (mol. wt. 50 kDa) and two identical light (L) chains (mol. wt. 25 kDa). Each chain is made up of domains of about 110 amino acids held together in a loop by a disulphide bond between two cysteine residues in the chain. The domains have the same basic structure and many areas of similarity in their amino acid sequences. The heavy chains determine the isotype of the immunoglobulin, resulting in pentameric IgM (Fig. 1.9), dimeric IgA (Fig. 1.10) or monomeric IgG.
Fig. 1.8 Basic structure of an immunoglobulin molecule. Domains are held in shape by disulphide bonds, though only one is shown. C1–3, constant domain of a heavy chain; CL, constant domain of a light chain; VH, variable domain of a heavy chain; VL, variable domain of a light chain. =S=, disulphide bond.
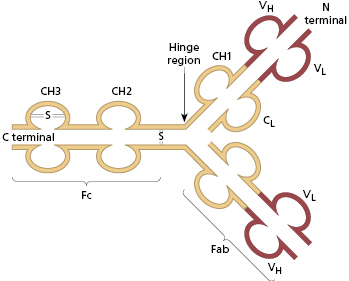
The amino (N) terminal domains of the heavy and light chains include the antigen-binding site. The amino acid sequences of these N-terminal domains vary between different antibody molecules and are known as variable (V) regions. Most of these differences reside in three hypervariable areas of the molecule, each only 6–10 amino acid residues long. In the folded molecule, these hypervariable regions in each heavy and light chain come together to form, with their counterparts on the other pair of heavy and light chains, the antigen-binding site (Fig. 1.8). The structure of this part of the antibody molecule is unique to that molecule and is known as the idiotypic determinant. In any individual, approximately 106–107 different antibody molecules could be made up by 103 different heavy chain variable regions associating with 103 different light chain variable regions, though there are even more epitopes due to further variation during the later processing (see section 1.4.1).
The part of the immunoglobulin chain next to the V region in either heavy or light chains is the constant (C) region; this is made up of one domain in a light chain (CL) and three or four in a heavy chain (CH) (Fig. 1.8). There are two alternative types of CL chain, known as kappa (κ) and lambda (λ); an antibody molecule has either two κ or two λ light chains, never one of each. Of all the antibodies in a human individual, roughly 60% contain κ and 40% contain λ light chains. There are no known differences in the functional properties between κ and λ light chains. In contrast, there are several possible different types of CH domain, each with important functional differences (Table 1.4). The heavy chains determine the isotype of the antibody and the ultimate physiological function of the particular antibody molecule. Once the antigen-binding site has reacted with its antigen, the molecule undergoes a change in the conformation of its heavy chains in order to take part in effector reactions, depending on the isotype of the molecule.
The processes by which the components of this supergene family are produced are identical for TCR and BCR and known as recombination. Immunoglobulin production, whether for BCR or antibody production, is the same initially. As for the TCR, the genes for the different chains in a BCR are carried on different chromosomes (Fig. 1.11). Like those coding for other macromolecules, the genes are broken up into coding segments (exons) with intervening silent segments (introns). The heavy chain gene set on chromosome 14 is made up of small groups of exons representing the constant regions of the heavy chains [e.g. mu (μ) chain] and a very large number of V region genes, perhaps as many as 103. Between the V and C genes are two small sets of exons, D and J (Fig. 1.11). In a single B cell, one V region gene is selected, joined to one D and J on the same chromosome; the VDJ product is then joined at the level of RNA processing to Cμ when the B cell is making IgM. The cell can make IgG by omitting the Cμ and joining VDJ to a Cγ. Thus, the cell can make IgM, IgD and IgG/A/E in sequence, while still using the same variable region. The same enzymes are used for the TCRs, and coded for by two recombination-activating genes control VDJ gene recombination: RAG1 and RAG2. Disruption of the RAG1 or RAG2 function in infants who have mutations in these genes causes profound immune deficiency, characterized by absent mature B and T cells, as neither TCR or BCR can be produced. On a different chromosome (either chromosome 22 for λ chains or chromosome 2 for κ chains) in the same cell, a V gene is joined to a J gene (there is no D on the light chain) and then the VJ product is joined at the RNA level to the Cκ or Cλ (Fig. 1.11).
The wide diversity of antigen binding is dependent on the large number of V genes and the way in which these may be combined with different D and J genes to provide different rearranged VDJ gene segments. Once V, D and J rearrangement has taken place to produce a functional immunoglobulin molecule, further V region variations are introduced only at a much later stage, when antibodies rather than BCRs are produced by the process of somatic mutation in germinal centres.
Natural killer cells also have recognition molecules. These cells are important in killing virally infected cells and tumour cells. They have to be able to recognize these targets and distinguish them from normal cells. They recognize and kill cells that have reduced or absent MHC class I, using two kinds of receptors [inhibitory (KIR) and activating (KAR)] to estimate the extent of MHC expression. They also have one type of Fc IgG (Fcγ) receptor, that for low-affinity binding of IgG antibodies, and so NK cells are able to kill some cells with large amounts of antibody on their surfaces. Further subsets of NK-like cells that contribute to innate immunity include NKT cells and invariant NKT cells (section 1.3.6); these are thought to be particularly important in tumour immunology (sections 1.5.1).
The major purpose of the complement pathways is to provide a means of removing or destroying antigens, regardless of whether or not these are coated with antibody. This requires that complement components recognize damaging material such as immune complexes (antigen combined with antibodies) or foreign antigens. The complement pathways are discussed in more detail in section 1.3.5.
1.2.3 Accessory molecules
The binding of a processed antigen–MHC class II complex on an antigen-presenting cell to the corresponding TCR provides an insufficient signal for T-cell activation; the binding of accessory molecules on the two cell surfaces provides additional stimuli. Accessory molecules are lymphocyte surface proteins, distinct from the antigen binding complexes, which are necessary for efficient binding, signalling and homing. Accessory molecules are invariant, non-polymorphic proteins. Each accessory molecule has a particular ligand – a corresponding protein to which it binds. These ligands are present on all cells which require close adhesion for functioning; for example, there are those on T cells for each of the many cell types that can activate or respond to T cells (antigen-presenting cells, endothelial cells, etc.); similar ligands are present on B cells for efficiency of T-cell help as well as stimulation by follicular dendritic cells.
There are several families of accessory molecules, but the most important appear to be the immunoglobulin supergene family of adhesion molecules, which derives its name from the fact that its members contain a common immunoglobulin-like structure. Members of their family strengthen the interaction between antigen-presenting cells and T cells (Fig. 1.12); those on T cells include CD4, CD8, CD28, CTLA-4, CD45R, CD2 and lymphocyte function antigen 1 (LFA-1). For interaction with B cells, CD40 ligand and ICOS are important for class switching (see section 1.4.3). Adhesion molecules, for binding leucocytes (both lymphocytes and polymorphonuclear leucocytes) to endothelial cells and tissue matrix cells, are considered in section 1.2.6. On B cells, such molecules include CD40 (ligand for CD40L, now named CD154) (Case 3.2), B-7-1 and B7-2 (ligands for CD28).
Fig. 1.12 Diagrammatic representation of adhesion molecules on T cells and their ligands on antigen-presenting cells/virus-infected target cells.
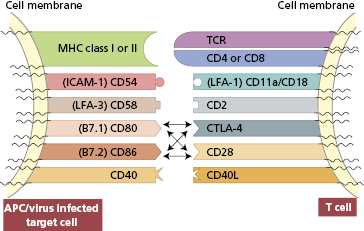
1.2.4 Effector molecules for immunity
There are humoral and cellular effector molecules in both the innate and the adaptive immune systems (Table 1.5). Several of the same mechanisms are used in both types of immune responses, especially in killing of target cells, suggesting that evolution of immune responses has been conservative in terms of genes, though with much redundancy to ensure the life-preserving nature of the immune systems in the face of rapid evolution of pathogenic microbes.
Table 1.5 Effector molecules in immunity
Innate | Adaptive | |
---|---|---|
Humoral | Complement components for opsonization or lysis | Specific antibodies for opsonization and phagocytosis or lysis with complement |
Cellular | Perforin in NK cells creates pores in target cell membranes | Perforin in cytolytic (CD8) T cells creates pores in specific target cell membranes, allowing entry of granzymes to cause apoptosis |
NKT cells induce apoptosis by perforin production | ||
Granzymes in NK cells induce apoptosis in target cells | ||
Lysosomes in phagocytic vacuoles result in death of ingested microbes | ||
Preformed histamine and related vasoactive substances as well as leukotrienes in mast cells |
Antibodies
Antibodies are the best described effector mechanisms in adaptive immunity. They are the effector arm of B cells and are secreted as soluble molecules by plasma cells in large quantities, to be carried in the blood and lymph to distant sites. As shown in Table 1.4, there are five major isotypes of antibodies, each with different functions (see also Box 1.2).
IgM is phylogenetically the oldest class of immunoglobulin. It is a large molecule (Fig. 1.9) and penetrates poorly into tissues. IgM has five complement-binding sites, which results in excellent activation of the classical complement pathway.
IgG is smaller and penetrates tissues easily. It is the only immunoglobulin to provide immune protection to the neonate (Table 1.4) as IgG is actively transported across the placenta. There are four subclasses of IgG, with slightly different functions.
IgA is the major mucosal immunoglobulin – sometimes referred to as ‘mucosal antiseptic paint’. IgA in mucosal secretions consists of two basic units joined by a J chain (Fig. 1.10); the addition of a ‘secretory piece’ prevents digestion of this immunoglobulin in the intestinal and bronchial secretions.
IgD is synthesized by antigen-sensitive B lymphocytes, is not secreted, acting as a cell-surface receptor for activation of these cells by the specific antigen relating to the BCR; it is essential for activation of antigen-responsive B cells.
IgE is produced by plasma cells but is taken up by specific IgE receptors on mast cells and basophils. IgE then provides an antigen-sensitive way of expelling intestinal parasites by increasing vascular permeability and inducing chemotactic factors via mast cell degranulation (see section 1.7).
IgM is a large molecule whose major physiological role is intravascular neutralization of organisms (especially viruses). IgM has five complement-binding sites, resulting in excellent complement activation and subsequent removal of the antigen–antibody–complement complexes by complement receptors on phagocytic cells or complement-mediated lysis of the organism (see section 1.4).
IgG
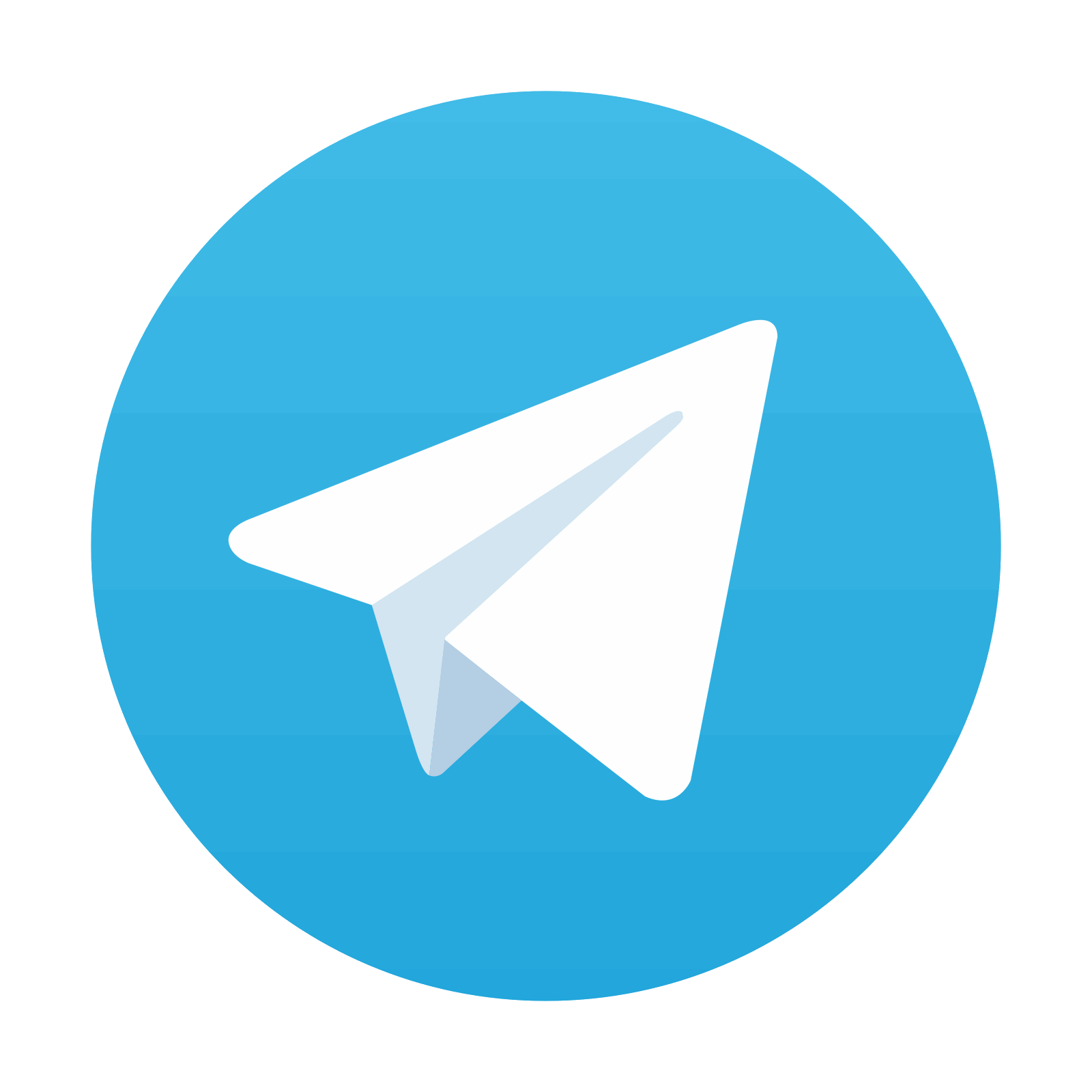
Stay updated, free articles. Join our Telegram channel

Full access? Get Clinical Tree
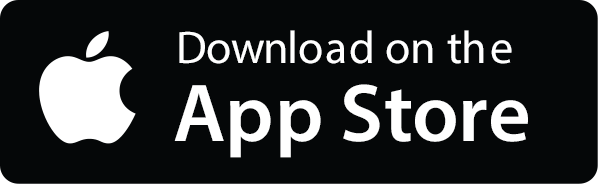
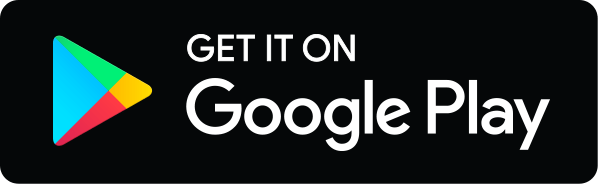