!DOCTYPE html PUBLIC “-//W3C//DTD XHTML 1.1//EN” “http://www.w3.org/TR/xhtml11/DTD/xhtml11.dtd”>
Basic Principles of Radiation Oncology
Deborah Citrin
As one of the three widely accepted modalities in the management of cancer, radiotherapy (RT) maintains perhaps the most “alternative” form; it is neither chemical nor invasive. Indeed, because of the general lack of understanding of the physics and biology underlying the principle and practice of RT, many clinicians and patients remain perplexed by its ability to cure disease and the constant evolution in its delivery to maintain the therapeutic window, ultimately designed to preserve quality of life and functionality while eradicating disease.
RADIATION BIOLOGY AND PHYSICS
Although radiobiology and physics are clearly separable in theory, and many textbooks have been written to focus on one or the other, a practical understanding of one subject cannot be easily delineated from the other.
Prior to discussing the biology and antineoplastic activity of RT, it is important to understand the basic physical properties of contemporary RT, in terms of both its activity and its production by a modern linear accelerator. The most common form of RT used today is a photon, or packet of x-rays. Discovered by Wilhelm Röntgen in 1895, photons were found to blacken photographic film; as these “new kinds of ray” were unable to be measured at the time, they were called “x-rays.” Shortly following their discovery, Wilhelm Freund treated a mole with the x-rays, giving birth in 1897 to the field of therapeutic radiology or radiation oncology. The following year, in 1898, Antoine Bequerel discovered radioactivity and the Curie family isolated radium. Indeed, because of the transportability and ease of administration, radioactive elements such as radium remained the preferred route of RT until only recently. Subsequent experiments demonstrated that the energy released by the radioactive decay of an element, so-called γ-ray, was identical to that artificially produced by a gas tube, an x-ray. Today, most modern RT consists of photons produced by linear accelerators, though there are many effective and curative applications from radioactive decay, generally termed brachytherapy.
Mechanism of Action
RT, in any of its forms, represents ionizing radiation because the energy passed from RT to the tissues is sufficient to cause an outer orbital electron to be released, thus ionizing or charging the tissue’s atom. Specifically, x-rays and γ-rays are forms of electromagnetic radiation, while the less commonly used forms of RT (protons, neutrons, mesons, carbon ions) are generally termed particulate radiation because they have mass. Despite the fact that RT “excites” or ionizes tissue particles, and thus can be measured by calories, it does not increase the temperature of the recipient or induce a thermal effect. So-called radiation burns are not thermal; the erythema of the skin is a reflection of the denudement of the superficial layers of the skin, caused by the increased cellular turnover induced by RT, leaving behind the better-vascularized deeper dermal tissues to show through as red.
When RT is delivered it is either directly or indirectly ionizing. Particulate, charged, or heavy ions deliver their energy to the tissue by direct effect; that is, these particles have enough kinetic energy to directly alter the genetic material with which they make contact. On the other hand, electromagnetic RT (γ- and x-rays) affect cells by indirectly ionizing them. These rays give up their energy to the absorbed tissues, thus causing the formation of fast-moving charged particles. Typically, the energy of an x-ray or γ-ray is passed to many circulating electrons (often called free radicals), which then go on to interact with various host tissues, including the genetic material of cancer cells. It is this interaction with the DNA of cancer cells that underlies the biology of RT.
Linear Accelerator
Modern RT is manufactured by a complex machine called a linear accelerator. The basic premise of this technology is that electrons are accelerated to a frequency of 3,000 megacycles per second and then are shot at a tungsten steel target. The negatively charged electrons are then repelled by the orbiting electrons of the steel target and, as they are deflected away and change direction, they lose some energy. In the observation of Newton’s laws regarding conservation of energy, the energy lost by the deflection of the electron interaction is gathered into a form, called an x-ray. The gathered x-rays (photons) are then shot out of the head of the linear accelerator into the patient. There are many beam-modifying devices (wedges, compensators, and blocks, among others) that can be placed between the accelerator and patient to conform the radiation to accomplish its goal of sparing normal tissues while targeting tumors.
Treatment Planning
In order to determine the methods and specifics by which the beam should be modified to accomplish its goals, the radiation oncologist works closely with several specialists. In fact, because of the complexity and ever-changing landscape of the field of radiation oncology, the days when the single clinician could consult a patient, set them up for treatment, calculate the physical parameters of the RT, and actually deliver the treatment are extinct. Many clinicians have been trained to operate all elements of the department, but this remains inefficient and the increasing volume of patients for whom radiation therapy is a necessary modality has relegated the clinician to consultation of the patient, delineation of the treatment target, prescription of the RT, and oversight of the various affiliated healthcare professionals responsible for the myriad of treatment responsibilities.
In today’s modern department, a simulator technologist sets the patient on a fluoroscopy unit or CT simulator to determine the site to be treated. The medical dosimetrist then takes the clinician’s prescription and assists in determining the most appropriate beam arrangement to accomplish the goals of therapy. The medical physicist, whose main responsibility is to ensure the machines are properly referenced and operating without any problems on a daily basis, calculates the dose delivered by the machine to coincide with the prescription without error. The radiation technologists then actually deliver the radiation therapy, closely following all set-up data provided to them by the physician, dosimetrist, and simulator technologist. The radiation technologist may also be responsible for performing ultrasounds, x-rays, or CT scans of the patient prior to each treatment fraction to ensure that the location receiving treatment is without error. The radiation oncology nursing team then evaluates the patient every few days of treatment to ensure there are no concerning side effects that require attention and they, along with the physician, initiate the appropriate clinical response. Furthermore, with the increasing realization that RT is often improved by sensitizing tumors with targeted agents and cytotoxic and cytostatic chemotherapeutics, the nurse and physician need to be more aware of potential interactions, toxicities, and tumor-response parameters than previously encountered when RT alone was delivered. Because of the evolving combined- or multimodality approach to many cancers, it is important to mention the pure biologic characteristics of tumors and normal tissues, to appreciate how and why RT alone has become less commonly used in the definitive and curative treatment of cancer.
FUNDAMENTALS OF RADIOBIOLOGIC PRINCIPLES
There are four fundamental radiobiologic principles that are considered by a radiation oncologist when determining the course of RT to be delivered: cellular repair, repopulation, redistribution, and reoxygenation.
Cellular Repair
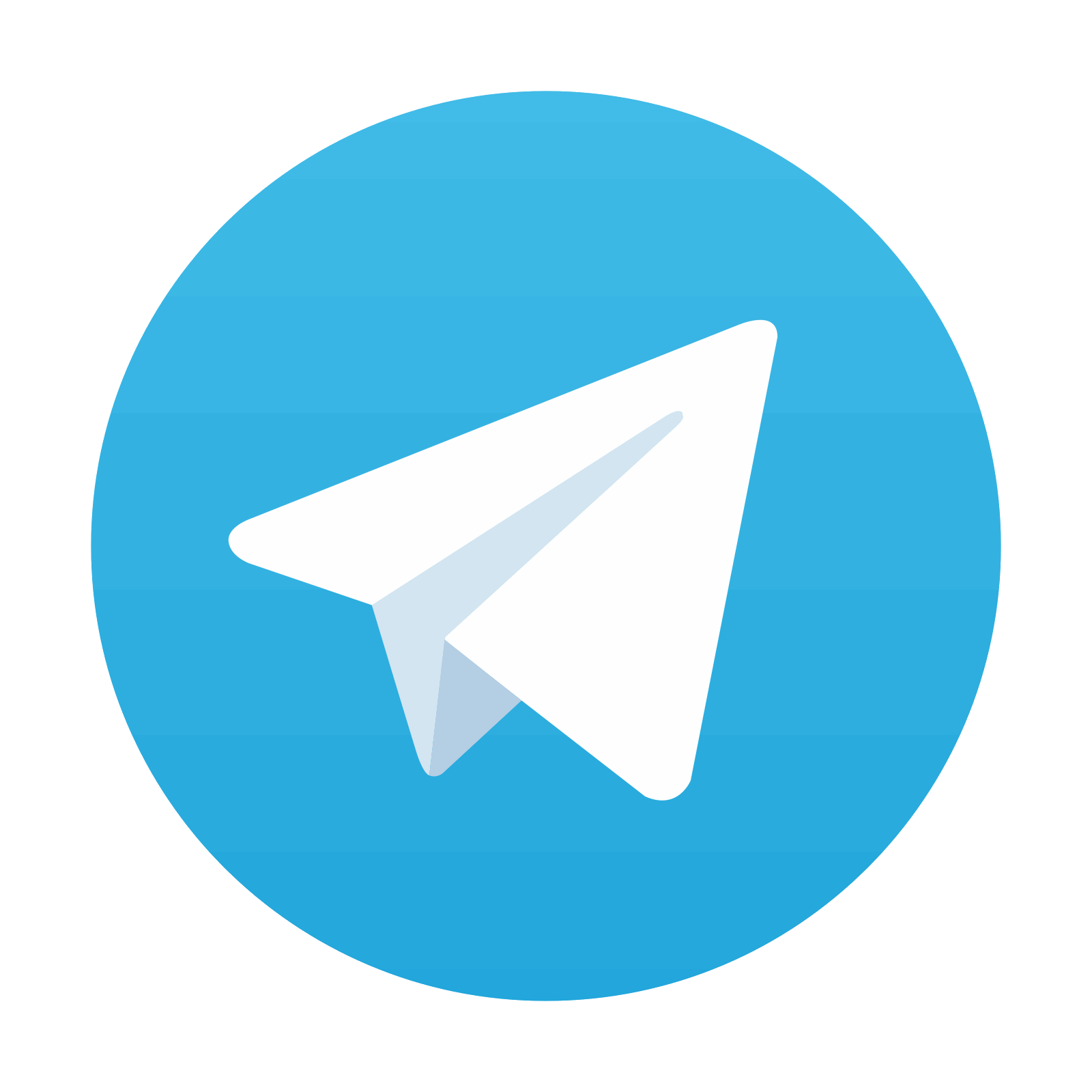
Stay updated, free articles. Join our Telegram channel

Full access? Get Clinical Tree
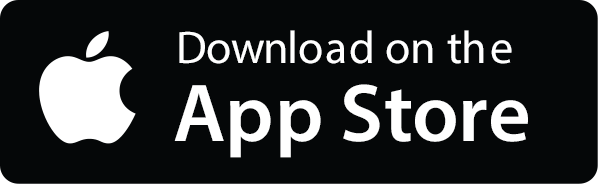
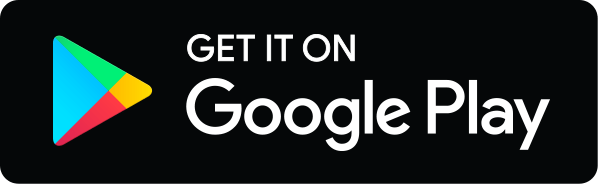